Research Journal of Applied Sciences, Engineering and Technology
Adsorption of Cobalt Ion from Aqueous Solution Using Biomaterial of Microalgae Oscillatoria sp Isolated from Teluk Jakarta
Research Journal of Applied Sciences, Engineering and Technology 2020 17: 7-12
Cite This ArticleAbstract
The adsorption of cobalt onto biomaterial of microalga Oscillatoria sp was investigated via batch experiment. Marine alga Oscillatoria sp was isolated from Teluk Jakarta Indonesia. The characterization of the functional group with FTIR spectrum showed microalgae have a functional group of $O-H$, $\>C = O$, $\>COO$, $\>C-O-C$, $\>N-H$, $\>C-S$, $\>C-H$ and $PO_4^{3-}$. Adsorption of cobalt ion was investigated as a function of time, pH, initial cobalt concentration, the dosage of biosorbent, salinity dan shaking. Equilibrium and kinetic adsorption were obtained from the batch experiment. The cobalt adsorption followed the Langmuir and Freundlich, isotherm models. The Freundlich constant (Kf) and n were $0.1186 \>(mg/g) (L.mol)^{\frac 1 n}$ and $8.43\>mg/L$, respectively. The maximum adsorption capacity was 4.71 mg/g at pH 8. Oscillatoria sp could adsorb cobalt even at a lower concentration, indicating a good affinity for metal. Kinetic studies showed that the adsorption of cobalt ion followed a pseudo-first-order with $k_1 \>= \>1.2×10^{-3} min^{-1}$.
Keywords:
Introduction
The heavy metal contaminant is a problem in the aquatic environment because it can be accumulated in the human body and toxic above the tolerance level (Al-Rub et al., 2004). Cobalt is one of the heavy metal that must be reduced in the aquatic system. The higher of $Co^{2+}$ solution was explored to remove $Co^{2+}$ from solution (He et al., 2011). High-level cobalt concentration caused DNA damage (Simonsen et al., 2012; Leyssens et al., 2017). One technique to reduce cobalt contaminants in the aquatic is by adsorption technique using biosorbent. Biosorption is adsorption and accumulation process of pollutants from aqueous solution using the biological materials. Biosorption technique has an advantage in reducing heavy metal ions to a very low level (Feng and Aldrich 2004). Many types of biomass have been studied for cobalt uptake as lemon peel (Bhatnagar et al., 2010), almond green hull (Ahmadpour et al., 2009) Blighia sapida (Jimoh et al., 2012).
Algae is one of the biosorbent in adsorb of toxic heavy metal because of it easy to grow and have a functional group in the wall cell. This functional group including carboxyl, sulfate and phosphate (Ahuja et al., 1999; Aravindhan et al., 2007). Many studies have shown that algae process high metal binding capacities (Deng et al., 2007). Oscillatoria sp, a kind of cyanobacteria microalgae, is widely distributed in eutrophic freshwater and marine environment. Several research has been used Oscillatoria as biosorbent to uptake of the heavy metal such as Cu and Zn absorption (Ahuja et al., 1997; Ahuja et al., 2001; Al-Shammary and Abdulhay 2016), Oscillatoria sp for adsorption of Cd (Azizi et al., 2012), Pb (Kumar et al., 2011).
In this study, we will explore the potential of microalgae Oscillatoria sp for the adsorb of cobalt (II) ion from aqueous solution. Marine microalga Oscillatoria sp was isolated from Teluk Jakarta, Indonesia. Effect of pH, adsorbent dosage, the concentration of cobalt, shaking and salinity will be performed in this study. Equilibrium isotherms for the adsorption of cobalt were measured experiment. Adsorption isotherms were determined with Langmuir and Freundlich equation.
Materials And Methods
Prepared the material: Microalgae Oscillatoria sp obtained from an Indonesian culture collection, LIPI Cibinong. It was grown at 25°C and pH adjusted to 8. The algae were harvest after 14 days of growth. Thereafter, the algal pellet was washed with deionization distillation water and then used for metal uptake experiment. Characterization of functional groups from the biosorbent using FTIR. The chemical reagents used in this study are analytical reagent grade. The cobalt solution was prepared by dissolving the $Co(NO_3)_2.6H_2O$ salt in distilled water. The initial ion concentration range 5-50 mg/L. The pH of the solution was adjusted by adding 0.1 M HCl or 0.1 M NaOH. The concentration of cobalt determined using an Atomic Absorption Spectrometer (AAS) (SHIMADZU A6000). Adsorption reading was taken in 240.7 nm.
Adsorption study: The metal uptake experiment was performed in 250 mL Erlenmeyer flask containing 25 mL Co 50 mg/L and 0.25 g biosorbent. The solution becomes to 50 mL with added of demineralization water. The experiment was carried out in batches in different condition of time, concentration, pH, amount of biosorbent, etc. The biosorbent with a known weight was left in contact with 50 mL of cobalt solution (5-50 mg/L) at 150 rpm. The mixture was shaken for 60 min. The parameter adsorption consist of variation of contact time (30-120 min), dosage of biosorbent (0.1-0.6 g), initial concentration of cobalt (5-50 mg/L), shaker (50-200 rpm), pH (3-8) and salinity (0-30 g/L). Thereafter supernatant liquid was filtered and the concentration of cobalt was determined by AAS. The adsorbed quantities of cobalt ion were evaluated using the following equation:
$ \text qe =\frac {(C_o-C_e)V}W $
where,
qe = The amount or concentration of cobalt adsorbed onto the unit amount of microalgae Oscillatoria sp (mg/g)
Co = The initial concentration of cobalt (mg/L)
V = The volume of solution (L)
w = The weight of biosorbent (Liu et al)., 2010; Jeppu and Clement, 2012)
The adsorption percentage of cobalt was adsorbed by biosorbent used the equation below:
$ \text Adsorption(\%) = 100 \frac {(C_o-C_e)}{c_e}$
where,
$C_e$ = Cobalt concentration in solution
$C_o$ = The cobalt initial concentration
Adsorption isotherms: The Langmuir and Freundlich models were studies for equilibrium state. The Langmuir equation was shown below:
$ \frac{C_e}{q_e} = \frac 1{q_{mon}.k_{l}}+\frac 1{q_{mon}}.Ce $
$ \ln q = \ ln K + \frac 1 n \ln C $
where,
$C_e$ = The solute concentration (mg/L) at equilibrium
$q_e$ = The amount of solute at equilibrium (mg/g)
q = Mon related to adsorption capacity
K = Langmuir constant
The Freundlich equation is an empiric model based on heterogeneous adsorption (Boudrahem et al., 2011):
$ \textit qe = k f C e^{1/n}$
$ \log q_{e} = \log Kf + \frac 1 n \log \textit Ce$
Where Kf is the Freundlich constant indicated the adsorption intensity.
Kinetic study: In a different measuring flask, 25 mL of cobalt 50 ppm (known concentration) and a known amount of adsorbent was taken periodic shaking. The solution was filtered and evaluated by atomic absorption spectrophotometer. The controlling mechanism of the metal adsorption process was studied by fitting first or second-order kinetic models. The linearity first-order kinetic model was shown in the below equation:
$ \log(qo-qt) = \log qe - \frac {k1}{2.003}\text t $
Where qt is the amount of adsorbate at time t (mg/g), qe is the adsorption capacity at equilibrium (mg/g) k1 is the pseudo-first-order rate constant $(min^{-1})$ and t is the contact time (minute). The linearity second-order kinetic model is given as:
$ \frac {t}{qt} = \frac {1}{k_{2}q^2} + \frac{1}{q_{e}} \textit t $
Where $k_2$ is the equilibrium rate constant of pseudo second-order adsorption $(g\;mg^{-1}. min^{-1})$.
Results And Discussion
Characterization of biosorbent: Characterization of biosorbent was done using FTIR analysis. FTIR spectra of biosorbent Oscillatoria sp is shown in Fig. 1. IR spectroscopy has been the method of choice for studying structure and interaction of molecules with identified of functional group (Banyay et al., 2003). The identification of functional group from Oscillatoria sp can be seen in Table 1. The stretching vibration of C-H indicated the methyl group and usually found in organic compounds (Coates 2006). The peak of C-H stretching usually occurs below 3000 cm-1. The peaks 1510-1450 is an aromatic stretch (C=C) and 850 cm-1 is C-H aromatic (Coates 2006). Biosorption of metal ion depends on the component and functional group of the biosorbent. This component including cellulose, oxygen, nitrogen, sulfur or phosphorus (Wang and Chen 2009). The most important of these groups including carboxyl, carbonyl, amine, amide, thiol and phosphonate (Volesky 2007).
Adsorption study: The cobalt sorption is depended on pH solution. The effect of initial pH on cobalt sorption capacity was studied at 25 mg/L cobalt initial concentration and the result is shown in Fig. 2. The uptake of cobalt in the pH range of 2-8 was determined in this experiment.
Figure 2 shows the relationship between pH and cobalt absorption efficiency by Oscillatoria sp. Biosorbent dosage of 0.25 g, the initial concentration of cobalt is 25 ppm and the volume of solution is 50 mL. An increase in pH caused an increase in the amount of cobalt absorbed. There is a linear correlation between pH and adsorption efficiency with a correlation of 0.9815 and equation $y = 17.263x-47.855$. The maximum efficiency is obtained at pH 8 with the adsorption of 23.58% and adsorption capacity of 4.71 mg/g. The pH solution can affect of metal-binding sites between cobalt and biosorbent. The cell wall ligands are closely associated with hydronium ion at the low of pH (Al-Rub et al., 2004). The increase of pH resulted in an increase in biosorption level because of influences of negative surface charge of the functional group. At the low pH occurred the competition between protons and metal ions in the adsorption process (Feng and Aldrich 2004).
Shaking is carried out to homogenize the solution and regulate the process of absorption of cobalt by Oscillatoria sp. The stirring range is carried out with variations of 50-250 rpm. The effect of shaking on cobalt adsorption by Oscillatoria sp can be seen in Fig. 3. Based on the experiment, the optimum stirring was obtained at 150 rpm with an adsorption efficiency of 41.81% and absorption capacity of 2.14 mg/g.
Variation of salinity ion solution from 0-30 g/L. Figure 4 shows the greater the salinity, the smaller of cobalt absorbed by Oscillatoria sp. The optimum absorption was obtained in salinity = 0 with optimum efficiency is 28.72% and absorption capacity of 1.43 mg/g. Analytical isotherms equation such as Langmuir and Freundlich isotherms are widely used for modeling adsorption data (Jeppu and Clement 2012). According to the Langmuir model, sorption occurs uniformly on the active site of the sorbent and once a sorbate occupies a site. These model isotherms can be seen in Fig. 5 to 7.
Figure 5 shows the effect of initial cobalt concentration on adsorption capacity onto Oscillatoria sp. The result showed a linear correlation between the initial cobalt concentration and absorption capacity with $R^2 = 0.976$ and equation $y = 0.1633x-0.5312$. The adsorption isotherm showed the adsorption molecule between the solid and liquid phase on the equilibrium state (Hameed et al., 2007). The applicability of the isotherm equation is compared with the correlation coefficient.
In the Freundlich model, the cases linear plots were obtained which reveal the applicability of this isotherm on cobalt adsorption process (Mittal et al., 2007). The condition experiment was done in pH = 5, the amount of adsorbent = 2.5 g and time of 60 minutes. The correlation shown linear with r = 0.9715 and equation of $y = 0.1186x + 0.1411$. The linear plot in Ce vs log qe shows that the adsorption of cobalt onto Oscillatoria sp follow the Freundlich isotherm model. The Freundlich adsorption isotherm was also applied for the adsorption of cobalt onto Oscillatoria sp. Freundlich constant kf and n were found to be $0.1186 (mg/g)(L.mol)^{1/n}$ and $8.43 mg/L$, respectively.
Optimum adsorption capacity (qm) biosorbent of cobalt to adsorb of cobalt according to Langmuir model was 6.24 mg/g. The slope and the intercept is 6.24 mg/g and 0,01 L/mg, respectively, that it indicated Q max and b. The Langmuir parameters can be used to knowing the affinity between the biosorbent and adsorbate. It can be used to suggests of the adsorption process is monolayer (Kalavathy et al., 2005; Ahalaya et al., 2010). The linear isotherm model is an alternative mathematical approach to predict the overall adsorption behavior (Chen 2015).
Kinetic of adsorption: The kinetic parameters were calculated by monitoring the effect of contact time, amount of adsorbent and concentrations of adsorbate solution on adsorption of cobalt. Figure 8 shows the effect of contact time on cobalt absorption by Oscillatoria sp. The increase in contact time shows the greater of cobalt absorbed. The optimum contact time is obtained by incubation for 120 min with an absorption capacity of 1.9338 mg/g. In can be inferred that the cobalt could ion could be adsorbed with a short time by using of Oscillatoria sp.
Figure 9 shows the effect of adsorbent dosage in cobalt adsorption by Oscillatoria sp. The algae dosage was 0.25 g and the initial concentration of $Co^{2+}$ ion was approximately 25 mg/L. The experiment shows increasing biosorbent dose increasing of cobalt adsorption in solution. The dose of biosorbent in the range of 0.1-0.6 g with the amount of adsorbent is 0.2983-0.3712 g. This adsorption involved in the mass transfer of ions from aqueous into the surface of biosorbent (Liu et al., 2010).
The kinetics of adsorption describes the rate of cobalt ions uptake on Oscillatoria sp microalgae and this rate control by the equilibrium time. The kinetic model of cobalt adsorption can be seen in Fig. 10. The kinetic model indicates the behavior of the adsorbent in the adsorption process (Aravindhan et al., 2007). The first-order rate, constant $(k_1)$ and qe were determined from the slope and intercept of the plot $(qo-qt)$ versus time contact. Kinetic studies showed that the adsorption followed a pseudo-first-order. The first-order rate constant $k_1$ can be found in the experiment was $1.2×10^{-3} min^{-1}$.

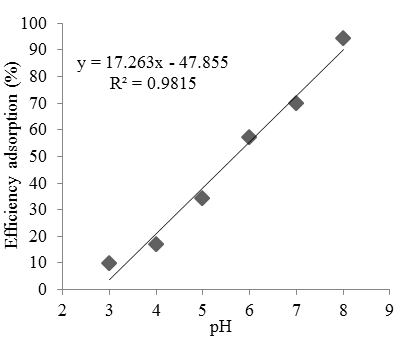
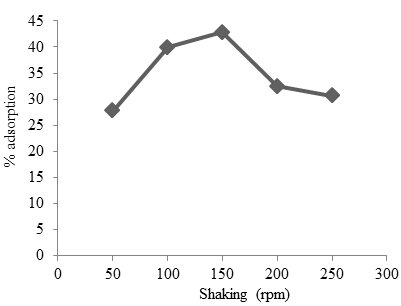
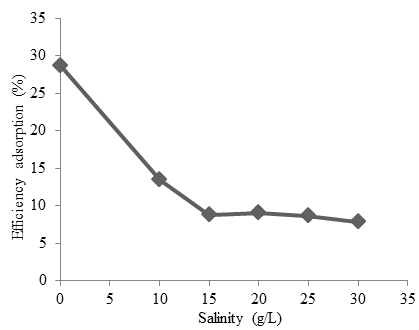
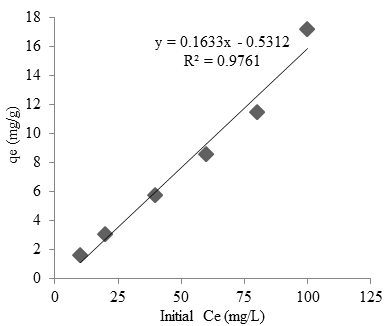
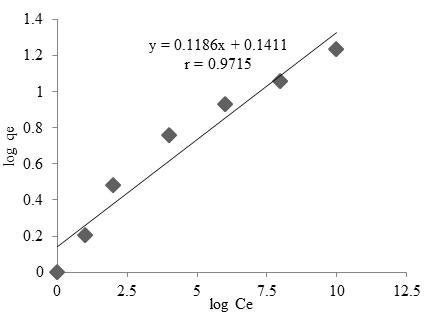
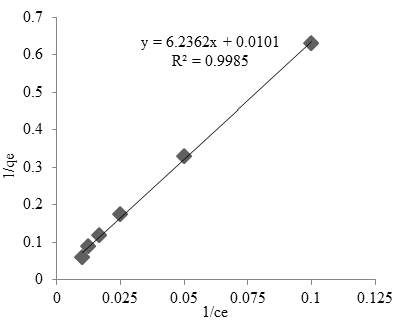
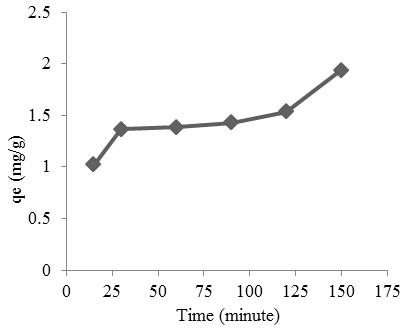
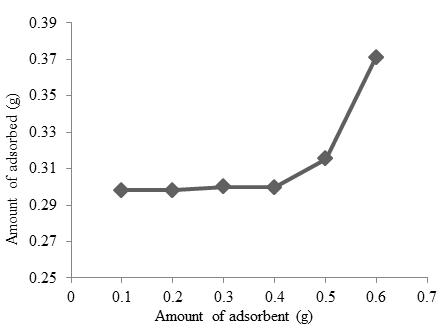
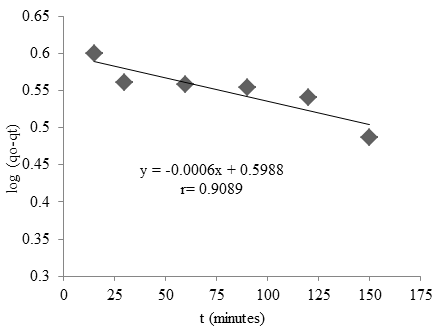
Wavenumber (cm-1) | Functional group | Chemical group |
---|---|---|
3272 | O-H | Alcohol |
2925 | O-CH3, C-H | Hydrocarbon |
2087 | C-H, N-H, C=O | Hyrocarbon, amine, carbonyl |
1634 | C-C | Hydrocarbon |
1515 | C-H | Hydrocarbon |
1449 | C-H, COO | Hydrocarbon, carboxylate |
1082 | C-N, C-O-C | Amine |
853 | PO43-, C-O-P | Phosphates |
596 | C-S, mineral | Sulfur, Anorganic compounds |
Conclusion
The experimental result shows that microalgae of Oscillatoria sp is an alternative for the removal of cobalt was depend on contact time, pH, adsorbent dosage and initial cobalt concentration. Analysis of functional group from biosorbent shows the peaks of hydroxide, carboxyl and carbonyl ions. This functional group is an important role in the biosorption of cobalt ion. The adsorption models of cobalt ion with Oscillatoria sp followed the Langmuir and Freundlich isotherms. The kinetic rate shows the pseudo-first-order.
Acknowledgement
This research received financial support from Politeknik AKA Bogor, Indonesia.
Conflict of Interest
No potential conflict of interest was reported by the authors.
Author Details
1Department of Analytical Chemistry, Politeknik AKA Bogor, Indonesia
2Department of Quality Assurance of Food Industry, Politeknik AKA Bogor, Indonesia
References
Rights and permissions
Open Access: This article is licensed under a Creative Commons Attribution 4.0 International License, which permits use, sharing, adaptation, distribution and reproduction in any medium or format, as long as you give appropriate credit to the original author(s) and the source, provide a link to the Creative Commons license, and indicate if changes were made. The images or other third-party material in this article are included in the article’s Creative Commons license, unless indicated otherwise in a credit line to the material. If material is not included in the article’s Creative Commons license and your intended use is not permitted by statutory regulation or exceeds the permitted use, you will need to obtain permission directly from the copyright holder. To view a copy of this license, visit http://creativecommons.org/licenses/by/4.0/
Cite this Article
DOI: http://doi.org/10.19026/rjaset.17.6028
Sections