Research Journal of Applied Sciences, Engineering and Technology
The Effectiveness of Heated Black Cotton Soil as a Low Calcium Pozzolanic Alumino-silicate Precursor in Lime-activated Stabilization of Black Cotton Soil for Use in Flood Prone Areas
Research Journal of Applied Sciences, Engineering and Technology 2020 17: 54-63
Cite This ArticleAbstract
The aim of this study was to investigate the effectiveness of heated black cotton soil as a precursor in lime-activated stabilization of black cotton soil in-place for use in flood-prone areas. Most methods used to improve expansive soils include the use of lime or lime-activated materials, cut to spoil followed by replacement and thermal treatment. Most lime treatment methods yield a material that cannot withstand high moisture levels and long flooding periods. Cut to spoil results in high costs and negative environmental consequences. Lime activated Ground Granulated Blast Furnace Slag (GGBS) has been found to be effective in flood conditions, but it has to be locally available. In this study, Unconfined Compressive Strength (UCS) tests were done on 50 mm diameter by 100 mm long stabilized soil specimens using various mixes of heated black cotton soil and lime which established an optimal mix of 14% heated black cotton soil at 400°C and 6% lime as the binders with 80% neat black cotton soil. Investigations of properties of this mix subjected to moist curing periods of 7, 14, 28, and 90 days followed by soaking them in water for 4 and 10 days after each curing period to simulate flash and severe flooding respectively were done. The durability reduction indices for each flooding scenario were computed. The mix yielded a strong durable product able to withstand flooding conditions. The heated black cotton soil at 400°C is, therefore, an effective low calcium aluminosilicate precursor with lime as the alkali activator.
Keywords:
Introduction
The presence of expansive soils greatly affects the construction activities in many parts of South-Western United States, South America, Canada, Africa, Australia, Europe, India, China and Middle East (Chen 1975). Black cotton soil, an expansive clay occupies about 3% of the world area, about 340 million hectares (Bhavsar and Patel, 2014).
Various methods that have been employed to improve expansive clays include mechanical compaction, surcharge loading, pre-wetting, lime stabilization, cement stabilization, fly ash stabilization, chemical stabilization and organic compounds treatment (Nelson and Miller, 1992). Other methods include cut to spoil followed by importing good material for replacement (Mokhtari and Deghani, 2012; Kalantari, 2012; Ministry of Transport and Communications (MOTC), 1987) and thermal treatment (Li et al., 2014; Wang et al., 2008; Terzaghi et al., 1996; Bell, 1993; O’Flaherty, 1974).
Cut to spoil followed by replacement with good materials and lime stabilization are among the more frequently used methods of dealing with black cotton soils (Mokhtari and Deghani, 2012; Kalantari, 2012; Yang and Zheng, 2006; MOTC, 1987). But excavating and importing good material for replacement has huge cost implications due to increase in carbon footage resulting from burning fossil fuel or cost due to damage to the environment resulting in increase in greenhouse gases, responsible for global warming (Prusinki and Bhattacharja, 1999; Yang and Zheng, 2006). Furthermore, good replacement materials put pressure on agricultural land since they are located in arable land which has led to legislation and imposition of levies in some countries for their excavation and transportation making them more and more expensive.
A number of industrial based bi-products and agricultural waste materials have been recently developed as stabilizers and the current emphasis is to amend these aluminosilicates with alkali activators such as lime in one-part geopolymer reactions to enhance their pozzolanic and cementitious properties (Luukkonen et al., 2018; Sharma and Sivapullaiah, 2016). The precursors that have been used include Ground Granulated Blast furnace Slag (GGBS), fly ash, silica fume, bagasse ash and rice husk ash with lime as the alkali activator (Kavak and Bilgen, 2016; Obuzor et al., 2012; Obuzor et al., 2011; Dang et al., 2016b; Wubshet and Tabese, 2014; Satyanarayana et al., 2016; Karatai et al., 2016). Only lime-activated GGBS has been found to be effective in withstanding flooding conditions (Obuzor et al., 2012). GGBS is not available in Kenya.
Thermal stabilization by heating or freezing has been used (Li et al., 2014; Wang Wang et al., 2008; Bell, 1993; O’Flaherty, 1974), but it’s a very expensive process. In-place improvement methods can result in natural resource conservation, reduction in energy usage, reduction in carbon dioxide emission and increased cost efficiency (Lee and Karunaratne, 2007; Phanikumar et al., 2008).
In Kenya, black cotton soil covers large areas and the solution has been to remove and replace them with suitable material. Structures and roads constructed in areas where black cotton soil is not removed crack extensively as the moisture levels change, with failures experienced depending on how extensive black cotton soil is. Thus, obtaining an effective local method of improving black cotton soil in-place will reduce the negative effects of black cotton soil and optimize on cost of construction. This study considers use of heated black cotton soil as a pozzolanic low calcium aluminosilicate precursor with lime as an alkali activator in a one-part geoploymer reaction in treatment of expansive black cotton soils in-place, to withstand flooding and high moisture conditions. According to Provis (2018), effectiveness of use of geopolymers is dependent on local availability of binders.
Materials And Methods
Materials: The target soil for investigation in this research was naturally occurring black cotton soil (NBC), an expansive clay. The aim of the study was to investigate the effectiveness of using lime-activated low calcium pozzolanic heated black cotton soil as a local in-place method of treating NBC to be used as a fill material for roads in high moisture conditions and areas subject to flooding, where suitable replacement fill material is not available. Wang et al., (2008) found that the highest temperature that heated expansive clay should be heated to lose swelling characteristics was, 600°C. Thus, in this research, mixes of Heated Black (HBC) soil only in temperature range 200-700°C and hydrated lime were used to stabilize the neat black cotton soil. Hydrated lime was preferred to quicklime because the latter is exothermic, extremely dangerous and capable of causing severe caustic burns (Mehta et al., 2014; O’Flaherty, 2002).
The NBC used as the expansive soil in this research was obtained from Mwihoko area of Kiambu County, about 25 km North East of Nairobi City in Kenya located 500 mm below ground. Basic soil tests to establish that the soil was expansive, were conducted in the Civil engineering laboratories of Jomo Kenyatta University of Science and Technology (Kenya) and at the Ministry of Transport, Infrastructure, Housing and Urban Design Materials Testing and Research Department (MTIHUDMTRD) laboratories in Nairobi, Kenya. The California Bearing Ratio (CBR), CBR swell, Unconfined Compressive Strength (UCS), specific gravity for the NBC soil determined in accordance with British Standards BS 1377 (1990), were 1%, 4.95%, 44.1 kN/m2 and 2.47 respectively. The liquid limit, plastic limit, plasticity index and linear shrinkage for the neat NBC soil were 65, 35, 30, 15 and 74%, respectively whereas compaction (proctor test T99) results revealed that the maximum dry density was 1272 kg/m3 at optimum moisture of 29.5% and air void content of 11%. The NBC was thus weak, had a high swell potential (O’Neil and Poormoayed, 1980) and was an expansive clay (Bhavsar and Patel, 2014).
X-ray diffraction (XRD) tests were done at the soils laboratories of the International Centre for Research in Agroforestry (ICRAF), Nairobi, for the NBC and HBC to obtain their mineralogy using a benchtop Bruker D2 Phaser diffractometer connected to a computer which had a software linked to International Center for Diffraction Data (ICCD), from which the mineralogy was inferred from the spectra obtained for each sample, based on Bragg’s Law. The results revealed that for each of the minerals, there were mainly 3 broad peaks for 2θ between 5° and 69° representing first, second and third order reflections corresponding to n values of 1, 2 and 3 from Braggs’ Law. The number of minerals varied from 5 to 7. The clay minerals and their percentages are shown in Table 1.
The swelling potential in Albite is mobilized if other factors influencing swelling such as presence of water and montomorillonite are fulfilled (Skippervik, 2014). In the soil under study, the presence of montomorillonite and water mobilized the swell potential due to the significant presence of the albite. The chemical tests done which included oxide content indicated that the calcium levels as CaO were low (less than 3%) for NBC and HBC and that they were pozzolanic agreeing with Greaves (1996) and Sherwood (1993) that clay minerals are natural pozzolans. However, pozzolanic action can only be manifested in the presence of water and calcium hydroxide.
The sulphates, chlorides, pH and loss on ignition determined in the MTIHUDMTRD laboratories were as shown in Table 2.
The highest sulphate content as $SO_4$ was 0.097% for HBC at 700°C. High levels of $SO_4$ in clays cause the $SO_4$ to react with $CaO$ for lime stabilized soils, to form ettringite $[Ca_6Al_2(OH)_{12}(SO_4)_3).27H_2O]$ or thaumasite $[CaSiO_3.CaCO_3.CaSO_4.14.5H_2O]$ which results in expansion and heave of the stabilized soil, causing distress in the structures built on the soil, which may lead to failure (Kavak and Bilgen, 2016; Beetham et al., 2015; Bell, 2007; Mitchell and Soga, 2005; Rajasekaran and Rao, 2005). $SO_4$ level in lime stabilized clays should therefore not exceed 0.25% (Beetham et al., 2015). Thus, the NBC and HBC at all temperatures could be used in lime stabilization without any risk of formation of ettringite or thaumasite.
The highest chloride level was 0.044% for HBC at 200°C; all the other levels were lower, for the NBC and HBC. The chloride level has no adverse effect because chlorides can be used to stabilize expansive clays, for levels of up-to 2% for sodium chloride. Where chlorides are used with lime, 1-5% sodium chloride can improve expansive soil with lime in the range 2-8% regardless of the percentage of lime (Davoudi and Kabir, 2011). The pH of the soil indicated a low alkaline level for the NBC and HBC at the tested temperatures. High levels of pH are desirable in lime stabilization. In lime stabilization, sufficient excess lime is added to ensure that the stabilized mixture maintains a pH value of at least 12.4 which is necessary to ensure the long-term stability of the hydration products (O’Flaherty, 2002; Dang et al., 2016a, et al., 2016b). There was progressive decrease in LOI due to combustion of organic matter. These results indicate that the NBC and HBC can be used in lime reactions without adverse effects.
Hydrated Lime was obtained from Athi River Mining of Nairobi.
Stabilization: Air dried NBC soil was stabilized with varying mixes of HBC soil and lime. A hand mixing process was adopted to ensure a homogeneous mix for a maximum stabilizer content of 20%. The specimens made were cylindrical 50 mm in diameter and 100 mm in length at moisture contents of 24, 28, 32 and 36%, respectively enveloping the optimum moisture content of the neat material.
Compaction was done in three layers and the specimen extruded with a metal plunger from a cylindrical mould, after which they were wrapped with cling film to regulate moisture movement. The mix ratios were as tabulated in Table 3. For each mix ratio, 16 specimens were made for each temperature of 200, 300, 400, 500, 600 and 700°C a total of 400 specimens. UCS tests were done in accordance with ASTM D559/D559M-15 (2015): Standard Test Methods for Wetting and Drying Compacted Soil-Cement Mixtures. The method is used to determine the resistance of compacted soil-cement specimen to repeated wetting and drying. This was done on each specimen after moist curing at room temperature for periods of 7, 14, 21, 28 days with samples wrapped in cling film to prevent rapid moisture loss.
From tests on these specimens, an optimum mix was obtained with the highest UCS value at an acceptable temperature of heated soil and lime content. Specimens were then made of the optimum mix which were subjected to various tests, making four specimens for each test.
There is a trend to use UCS as an alternative to CBR as the default test for strength for road works (Thomas, 2001) since UCS is better than CBR as a strength test because CBR being a penetration method is not good for soils taking tension as is the case in roads (Bandara et al., 2017). Further, the CBR tests also use too much material (Thomas, 2001). Thus, the strength tests for the trial mixes and the optimum mix for the various curing regimes were done in terms of UCS, to British Standards BS 1377 (1990). However, since the 7-day cure 7-day soak CBR test is the standard test for stabilized materials, it was done for the optimum mix in this research to compare the results with strength requirements in Kenya which are still in terms of CBR (MOTC, 1986; MOTC, 1987). Compaction characteristics and CBR were done to British Standards BS 1377 (1990).
Long term curing and simulated flooding regimes: UCS and physical properties comprising grading and Atterberg limits (British Standards BS 1377, 1990), free swell according to the method proposed by Holtz and Gibbs (1956) and XRD were tested after moist curing at room temperature for periods of 7, 14, 28, 56 and 90 days, with samples wrapped in cling film to prevent rapid moisture loss (Fig. 1) to investigate effect of long-term curing.
To simulate different flooding scenarios, UCS tests were done for specimens partially soaked in water for 4 and 10 days to simulate a normal flood and fully soaked/submerged for 4 and 10 days to simulate severe flooding, after each of the moist curing periods (Obuzor et al., 2011; Obuzor et al., 2012). Hossain et al., (2006) considered a moist curing period of 84 days followed by immersion in water for 7 days. The simulation by Obuzor et al., (2011) and Obuzor et al., (2012) was adopted since it represents a more severe scenario. The partial soaking was achieved by immersing 10 mm of the specimen in water.
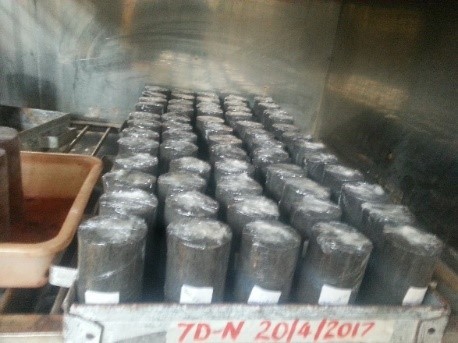
Soil sample type (HBC in °C) | ||||||||
---|---|---|---|---|---|---|---|---|
Reference Mineral | NBC | HBC at 200 | HBC at 300 | HBC at 400 | HBC at 500 | HBC at 600 | HBC at 700 | HBC at 800 |
Albite | 26.2 | 31.2 | 30.1 | 31.4 | 28.3 | 35.3 | 26.3 | 25.5 |
Microcline | 38.5 | 30.2 | 31.7 | 34.3 | 33.9 | 39.1 | 36.6 | 30.8 |
Kaolinite | 1.7 | - | - | 2.5 | 3.2 | 2.5 | - | 2.0 |
Montomo-rillonite | 3.3 | 5.8 | 7.1 | 4.8 | - | - | - | - |
Muscovite | 2.7 | - | - | 4.2 | 4.2 | 5.1 | 5.0 | 5.6 |
Calcite | 5.8 | 5.3 | 4.5 | - | 5.8 | - | - | 9.5 |
Imogolite | - | 3.3 | 4.0 | - | - | - | 2.5 | - |
Heat soil at temperatures stated in °C | |||||||
---|---|---|---|---|---|---|---|
Reference | Neat soil | 200 | 300 | 400 | 500 | 600 | 700 |
SO3(%) ×10-2 | 6.6 | 0.30 | 0.66 | 3.9 | 1.7 | 2.8 | 8.1 |
SO4(%) ×10-2 | 7.92 | 0.36 | 0.72 | 4.68 | 2.04 | 3.36 | 9.72 |
Cl (%) | 0.01 | 0.044 | 0.043 | 0.011 | 0.032 | 0.011 | 0.034 |
pH | 7.42 | 7.19 | 7.2 | 7.35 | 7.23 | 7.17 | 7.0 |
LOI (%) | 12.8 | 11.8 | 10.2 | 10.1 | 9.2 | 7.22 | 6.45 |
SO3 - Sulphate (III) Oxide; SO4 - Sulphates (IV) Oxide; Cl - Chloride Content; LOI - Loss on Ignition |
Neat material (NBC) | Heated soil (HBC) | Lime |
---|---|---|
80 | 20 | 0 |
80 | 18 | 2 |
80 | 16 | 4 |
80 | 14 | 6 |
80 | 12 | 8 |
Results And Discussion
Test results for trial mixes: The neat black cotton soil (NBC) was maintained at 80% for all mixes and thus the references were called out only in terms of the stabilizers’ mixes, while the binder proportions were varied. Thus mix 16-4 means 16% HBC and 4% lime as stabilizers. Max values were obtained at 36% compaction moisture content, due to the high-water demand for lime hydration. Peak values were obtained from mixes of 16-4, 14-6 and 12-8 for all the temperatures. Plots were therefore done for these mixes for each temperature of HBC, as shown in Fig. 2.
The highest UCS values were established as 264.5, 398 and 333 kN/m2 for mix 16-4, 14-6 and 12-8 with HBC at 400°C respectively and 458 kN/m2 for mix 12-8 with HBC at 600°C. Relatively low UCS values were obtained for HBC at 200°C, 300°C, 500°C and 700°C. This was very consistent for all the mix ratios. Though the highest strength was 458 kN/m2 for mix 12-8 with HBC at 600°C, it was disregarded because of the high temperature of HBC and the fairly high lime content. Therefore, the optimal mix was selected as 14-6, with a strength of 398 kN/m2 for a temperature of HBC at 400°C and lime content of 6%. Generally, strength increases with increase in lime content for lime-stabilized soils up-to a certain level, after which the strength decreases or remains constant with addition of lime (Bell, 1996; O’Flaherty, 2002). A maximum UCS value of 360 kN/m2 was obtained at lime content of 6.2% with lime as the only stabilizer for 7 days soak and 7 days cure. The strength of the optimal mix was 12% greater than this and will therefore perform better as a fill material.
Properties of the optimal mix: These are compaction, CBR, UCS, grading, swelling characteristics and XRD.
Compaction test results: The compaction results for the optimal mix are illustrated in Fig. 3.
The Maximum Dry Density (MDD) was 1348 kg/m3 with an optimum moisture content of 30%, at about 7.5% air voids, compared to NBC values which were 1272 kg/m3, 29.5% and 11% respectively.
The specific gravity was 2.58 which was an increase of 10% from the value for the neat material which was 2.47. Thus, the stabilizing improved the packing together of the soil particles. A highly permeable lime stabilized material can potentially lose strength through softening and removal of the pozzolanic products (McCallister and Petry, 1992; Le Runigo et al., 2009). Compaction should therefore achieve a minimum air voids value throughout the fill (Sherwood, 1992). Ingress of water in a highly permeable material causes the calcium silicate hydrates (C-S-H) to de-constitute into $Ca(OH)_2$ and silicate when the water is of neutral pH and $Ca^{2+}$ levels are low, raising the pH and $Ca^{2+}$ levels. CSH gel continues to de-constitute with the supply of more water (Taylor, 1990). In this study, the reduction of air voids by 32%, increase of specific gravity and MDD by 10% and 6% respectively gave a less permeable material, with a high durability suitable as fill material in high moisture conditions.
CBR results: The CBR increased from 1% for the NBC to 39.6% for the lime-HBC-NBC mixture, a percentage increase of 3860%, while the CBR swell reduced from 4.95 to 0.22%. The lime-HBC-NBC stabilized soil under test was class S6, with the CBR value exceeding the minimum required value of 30% for this class, with a negligible swell value. Thus, the stabilized material is suitable as a fill and subgrade material. The higher the subgrade strength, the more economical are the sub-base and base layers for any desired pavement structure (MOTC, 1987).
Effect of long-term curing and simulated flooding regimes on strength: The effect of long term curing and simulated flooding regimes was investigated by soaking the cured samples in water for specified periods and then taking the UCS value at the end of these periods. The durability of the stabilized soil was measured in-terms of the Strength Reduction Index (SRI) which is an empirical way of expressing the strength of a soaked material as a percentage of the same material in its un-soaked state. It is also called the durability index (Obuzor et al., 2012; Hossain et al., 2006; Thomas, 2001). The results for partial and full soakage regimes which simulate a normal and a prolonged flood are illustrated in Fig. 4 and 5 respectively for 4- and 10-days soaking periods. The 4- and 10-days soaking periods simulate the effect of a flash flood and a severe flood respectively.
As illustrated in Fig. 4, the 4 days partial-soak SRI values were above 100%, up-to 56 days of curing and then dropped to 70% at 90 days. This reflects a very high level of stability of the stabilized soil. The partial saturation ensured gradual availability of water for the hydration process, enhancing the curing process. The 10 days partial-soak SRI values were above 100%, up-to 28 days of curing and then dropped to 83% and 62% for 56 and 90 days curing respectively. They were generally below the values for the 4 days soak, except for the 28 days cure which was 22% higher. This represents a high level of stability up-to 28 days of curing, with a drop to 83% and 62% for 56 and 90 days respectively. The normal severe flood therefore has a more severe impact than the normal flash flood. Despite this the lowest strength recorded for a normal flood scenario was 75 kN/m2 at 56 days curing, which is strength of a Kenyan red soil which has UCS values in the range 75-100 kN/m2 and is used for fill and subgrades where it is available. It is worth noting that the strength recovered and became 139 kN/m2 at 90 days. Most road construction projects have construction projects exceeding one year and therefore by the time the road is fully loaded, the strength of the fill and subgrade will have recovered even if the road were to experience flooding during the construction period.
Therefore, the stabilized soil is suitable for use as a fill and subgrade material for road construction for flash floods, without risk of collapsing. The best curing period for normal flooding is 28 days which increased the strength by 35% and 57% for the 10 days and 4 days partial soak respectively.
As illustrated in Fig. 5, the 4 days submerged samples had SRI values higher than 100% for 7- and 28-days curing, while SRI values decreased steadily from the 28, 56- and 90-days curing period. Except for the 14- and 90-days cure, the SRI values for the 4 days soak were lower than for the 10 days soak. The latter were basically 100% and over except for the 90 days cured sample which was 40%. The UCS for this latter scenario was 89 kN/m2 which is a good strength for fills and subgrade. The lowest strength for full submergence was 73 kN/m2 for 56 days cure and 4 days soak. However, there was good recovery and the 90 days cure, 4 days soak gave a strength of 158 kN/m2.
The stabilizing thus produced a strong durable material, useful for fills in roads, buildings and other lightly loaded structures and also as a subgrade material for roads. The material withstood the worst simulated environment of severe prolonged flooding.
In both the normal and severe flooding regimes, there was a general trend of decrease in strength as the curing period increased as illustrated in Fig. 4 and 5. The drop-in strength at 56 days curing was a maximum of 19% which is considered not too significant, because the stabilized soil needed adequate moisture to satisfy the moisture demand for completion of the lime and lime-heated soil activated pozzolanic and hydration reactions. The greatest reduction was 40% for 90 days cured severe prolonged flooding scenario. According to Obuzor et al., (2012) in lime-activated Ground Granulated Blast furnace Slag (GGBS) stabilized soil, SRI values increase up-to 56 days, after which they start decreasing because the active period of moisture demand approaches its ultimate at 56 days when the hydration reaction nears completion. Further addition of moisture causes water to enter into the void spaces, causing a deleterious effect of reduced strength. At this point, the free lime and pH are in short supply. In this study, the same tread is apparent. The active period for moisture demand was ultimate at period of 56 days when the strength stabilized and hydration apparently had reached completion. However, it may not be practical to allow for a long curing period on site. Wild et al., (1998) suggested a moist curing period of 28 days before the stabilized fill material gets saturated with water, which agrees very well with the results of this study where the maximum loss of strength was 14% for the 28 days cured 4 days soak severe flooding regime.
Generally, the pozzolanic products of lime stabilization are Calcium-Silicate-Hydrate (CSH), Calcium-Aluminate-Silicate-Hydrate (CASH) from hydration and pozzolanic reaction (Das, 2016; Dang et al., 2016a), with CSH being the principal cementitious product resulting in enhanced strength of the stabilized material (Saravanan et al., 2017; Kavak and Bigen, 2016; Obuzor et al., 2012; Wild et al., 1999; Sharma and Sivapullaiah, 2016). These products contribute to the flocculation process by bonding adjacent soil particles together into larger-sized aggregates which strengthen the soil as curing occurs. However, where free lime and $Ca^{2+}$ and pH are in short supply especially when water enters the voids of stabilized soil particles, the CSH de-constitutes into $Ca(OH)_2$ and silicate (Taylor, 1990). Although this raises the pH and $Ca^{2+}$, with the supply of more water the CSH gel will continue to de-constitute, explaining why the strength dropped for curing periods of 56 and 90 days in flooding scenarios. This is what happened to the lime-activated heated soil-black cotton mixture in this research. The pozzolanic properties of the heated soil had the same effect as the GGBS, although GGBS is a calcium rich alluminosilicate, compared to the HBC at 400°C which is a low calcium pozzolanic alluminosilicate. Therefore, the heated soil at 400°C is effective as a low calcium pozzolanic alluminosilicate precursor in stabilization of black cotton soils, which aided in formation of more CSH gel and change in micro-pore orientation, forming a strong durable material for use as a fill material for roads and ground floor slabs and also for use as a subgrade. Ideally the stabilized soil should be moist cured for 28 days before exposure to flooding. These 28 days period gave the higher SRI values for normal flooding, with hardly any change between the 28- and 56-days cure for the prolonged flooding.
Grading/particle size distribution: The grading test results are shown in Fig. 6, for moist curing periods of 7, 14, 28, 56 and 90 days respectively. Fine soils have at least 35% by mass finer than 0.06mm while coarse soils have less than 35% finer than 0.06 mm. The samples cured for 7, 28, 56 and 90 days were fine, whereas the samples cured for 14 days were coarse. The first process that occurs when hydrated lime is added to a clay is lime modification followed by stabilization. Modification is a rapid cation exchange process which forms cementitious products in a flocculation-agglomeration process, transforming the clay into a more silt-like or sand-like material which has improved plasticity, swelling, workability and stability resulting into a better fill material (O’Flaherty, 1974; O’Flaherty, 2002; Dang et al., 2016a).
The combined silt and sand content obtained in the stabilized soil was above 50%, as a result of modification; the maximum clay content was 24% at 28 days cure, a reduction of 31% which gave a better fill material, with improved swelling characteristics due to reduced influence of the clay fraction. Silts, sands and gravels are normally non-plastic (Mitchel and Soga, 2005). The plasticity was established from Atterberg limits as discussed below, because grading is not a measure of plasticity.
Atterberg limits and free swell for varying curing periods: The swelling characteristics of the stabilized soil are shown in Fig. 7. The liquid limit (WL), the plastic limit (Wp), the plasticity index (Ip) and the Linear Shrinkage (LS) experienced very minor changes during the whole 90 days curing period, while the Free Swell (FS) decreased steadily.
In the curing periods of 7, 14, 28, 56 and 90 days respectively, the key index property of Ip dropped from 30 to 25%, while the WL dropped from 68 to 64% from 7 days to 90 days of curing respectively. These results indicate that the swelling properties of the stabilized black cotton improved during long term curing.
The lime-HBC-NBC system behaviour is apparently very similar to behaviour of a lime stabilized expansive clay, which causes cation exchange by replacing the monovalent ions adsorbed on the clay surface with the divalent $Ca^{2+}$ in the lime, reducing the diffuse double layer thereby causing attraction between the clay particles forming flocs with reduced swelling properties, during the modification stage.
According to O’Neil and Poormoayed (1980), suitable fill material for road construction should have a marginal swell potential, with WL ranging between 50-60% and Ip between 25-35%. However, MOTC (1987) limits the Ip to a maximum of 20 for stabilized material, without a qualification on WL. Based on these two requirements, the stabilized soil can be classified as being of marginal swell potential and thus suitable as fill material for road construction; the Ip is only nominally above 20% while the negligible value of CBR swell of 0.22% noted above affirms a non-swelling product. The linear shrinkage remained below 20% throughout the curing period, whereas the FS dropped to below 50% after 28 days curing. Lightly loaded structures such road pavements, residential buildings and canal linings suffer most damage when built on expansive soils (Chen, 1988). For free swell values below 50%, there is no appreciable volume change during wetting or drying even under light loads (Holtz and Gibbs, 1956). The formation of CSH, CAH and CASH during the curing process as noted earlier gave a strong and durable material (Dang et al., et al., 2016a; Das, 2016; O’Flaherty, 2002) suitable as a fill material for road construction and other light structures such as ground floor slabs.
Classification for varying curing periods: The classification was done as for the stabilized material based on the British System Classification System (British Standards BS 5930, 1990). The results are shown on Table 4, for the various curing periods.
The soil after stabilizing was either a silt or a sand of high plasticity. The modification process in stabilization transformed the clay into a more silt-like or sand-like material which improved plasticity, swelling, workability and stability resulting into a better fill material (O’Flaherty, 1974; O’Flaherty, 2002; Dang et al., 2016a).
Although the stabilized soil is classified as of high plasticity it has a marginal swell potential, with negligible CBR swell and therefore suitable as fill material for road construction.
XRD Properties: The percentages of the clay minerals were as shown in Table 5 for the various curing periods.
There were three broad peaks for 2θ between 5 and 62° while the number of minerals varied from 5 to 7. The main clay minerals were albite and microcline, whose percentage sum by weight were 49.5, 48.8, 50.7, 55.8 and 58.8 for the samples cured at 7, 14, 28, 56 and 90 days respectively. The percentages for the NBC are shown in Table 1, which shows that the percentage of albite and microcline were 26.2 and 38.5 respectively, giving a total of 64.7%. The montmorillonite increased from 3.3% for the neat to 6.6, 10.1, 8.4, 4.6 and 5.9 % for the 7, 14, 28, 56 and 90 days curing respectively. The most significant increase was for the calcite, which increased from 5.8% for the neat soil to 16, 15.4, 19.5, 15.1 and 14.5% for 7, 14, 28, 56 and 90 days curing respectively. Calcite is mainly calcium carbonate, which formed due to cementitious reactions (Solanki and Zaman, 2012). Despite the relative increase in the montmorillonite and the presence of albite, the soil remained non-swelling as indicated by the low CBR swell value of 0.22% accompanied with a high strength value as measured by CBR, which was 39.6% for the 7 days cure and 7 days soak indicating high strength stability.
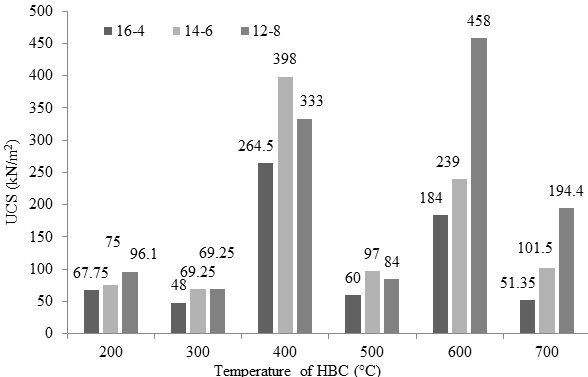
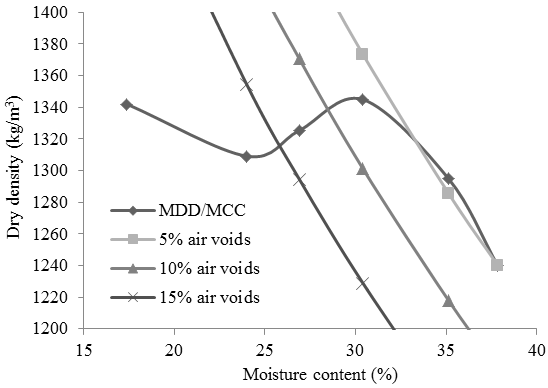
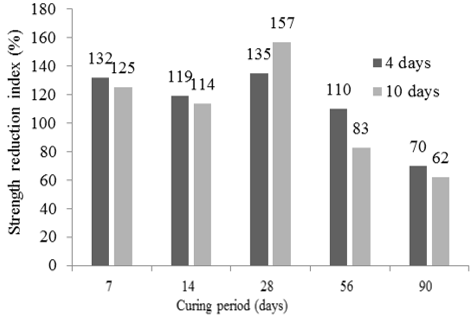
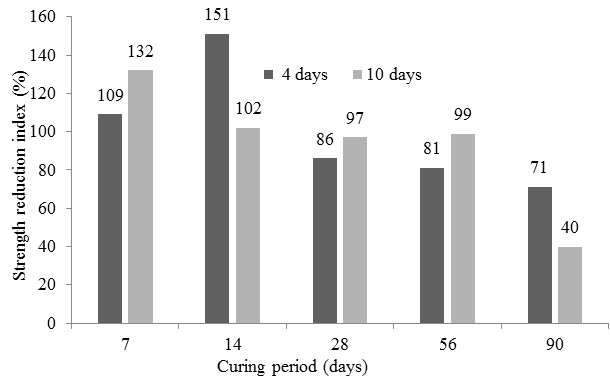
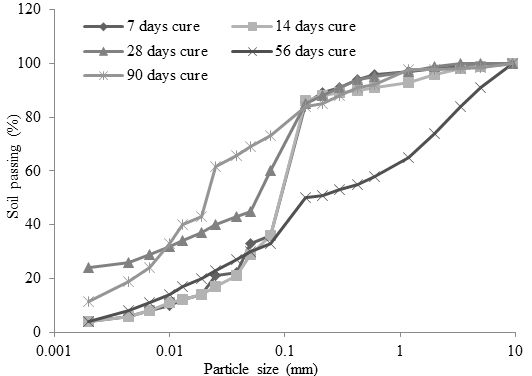
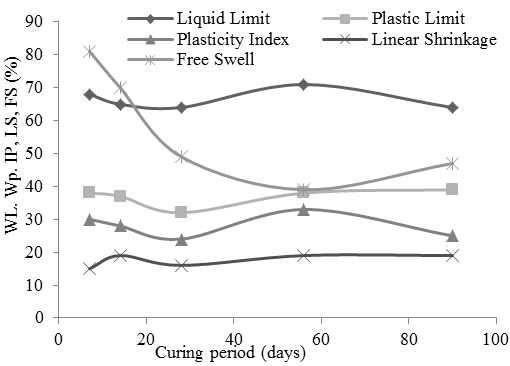
Curing period (days) | Description | Symbol |
---|---|---|
7 | Gravelly SILT of high plasticity | MHG |
14 | Very silty SAND of high plasticity | SMH |
28 | Gravelly SILT of high plasticity | MHG |
56 | Very clayey SAND of high plasticity | SCH |
90 | Sandy SILT of high plasticity | MHS |
Curing period (days) | |||||
---|---|---|---|---|---|
Mineral | 7 | 14 | 28 | 56 | 90 |
Albite | 24.9 | 21.2 | 21.7 | 18.6 | 23.9 |
Microcline | 24.6 | 27.6 | 29.0 | 37.2 | 34.9 |
Montmorillonite | 6.6 | 10.1 | 8.4 | 4.6 | 5.9 |
Calcite | 16.0 | 15.4 | 11.5 | 15.1 | 14.8 |
Imogolite | 5.1 | 5.9 | 0 | 3.4 | 3.3 |
Kaolinte | 0 | 0 | 1.3 | 2.1 | 0 |
Conclusion
- An optimal lime-activated stabilizer for black cotton soil with low calcium pozzolanic allumino-silicate heated black cotton soil heated at 400°C as the precursor was obtained, comprising 14% heated black cotton soil at 400°C and 6% lime. The stabilized black cotton is able to withstand harsh full soaking period of 10 days, representing a prolonged severe flood. Though the durability decreased with increasing curing period, the final product was acceptable as a fill and subgrade material at 90 days curing and 10 days soaking.
- The stabilized black cotton soil resulted into a very strong, non-swelling material with a very high soaked CBR of 39.6% and a CBR swell 0.22% giving a class S6 subgrade, suitable as a fill and subgrade material for roads and as a fill for ground floor slabs and other lightly loaded structures.
- The HBC at 400°C behaves in a similar manner to GGBS mixture in the stabilization process. GGBS is not available in Kenya since Kenya does not smelt iron ore. Thus, the HBC at 400°C is a suitable locally available low calcium aluminosilicate precursor for lime activation, in one-part geoplymer reactions whose effectiveness depends on local availability of binders (Provis, 2018).
- The use of the HBC at 400°C-lime mixture in-place will reduce negative environmental effects of dumpsites, reduce pressure on arable agricultural land as a source of fill material and reduce carbon dioxide emission in haulage of fill materials as well increase cost efficiency.
Acknowledgment
We acknowledge with gratitude the funding received from Jomo Kenyatta University of Agriculture and Technology (JKUAT). We are also thankful for the support given by the Sustainable Materials Research and Technology Centre (SMARTEC), the Department of Civil, Construction and Environmental Engineering both of (JKUAT), the Ministry of Transport, Infrastructure, Housing & Urban Design Materials Testing and Research Department (MTIHUDMTRD) laboratories in Nairobi and the soils laboratories of the International Centre for Research in Agroforestry (ICRAF), Nairobi for availing their facilities for material testing.
Author Details
1Sustainable Materials Research and Technology Centre, Jomo Kenyatta University of Agriculture and Technology (JKUAT), P.O. Box 62000-00200,
2Department of Civil and Construction Engineering, University of Nairobi, P.O. Box 30197, GPO, Nairobi, Kenya
3Department of Civil and Construction and Environmental Engineering (JKUAT), Kenya
References
Rights and permissions
Open Access: This article is licensed under a Creative Commons Attribution 4.0 International License, which permits use, sharing, adaptation, distribution and reproduction in any medium or format, as long as you give appropriate credit to the original author(s) and the source, provide a link to the Creative Commons license, and indicate if changes were made. The images or other third-party material in this article are included in the article’s Creative Commons license, unless indicated otherwise in a credit line to the material. If material is not included in the article’s Creative Commons license and your intended use is not permitted by statutory regulation or exceeds the permitted use, you will need to obtain permission directly from the copyright holder. To view a copy of this license, visit http://creativecommons.org/licenses/by/4.0/
Cite this Article
DOI: http://doi.org/10.19026/rjaset.17.6039
Sections