Advance Journal of Food Science and Technology
Incorporation of Hydrocolloids and Aloe Vera Gel on Tree Tomato Beverages (Cyphomandrabetacea S.) Part II: Physicochemical Properties and Colloidal Stability
Advance Journal of Food Science and Technology 2019 17: 28-36
Cite This ArticleAbstract
The aim of this study was to assess the effect of the incorporation of hydrocolloids and aloe vera on the physicochemical properties and the degree of stability of Tree Tomato Beverages (TTB). The addition of hydrocolloids was used to control the phenomenon of phase separation in TTB during storage. Concentrations of Xanthan Gum (XG) and carboxymethylcellulose (CMC) $ \ge $ 0.05% were appropriate in beverage physical stability due to the reduction in sedimentation velocities and high values of zeta potential (ζ>30 mV), without significantly affecting the physicochemical properties and color parameters. The incorporation of aloe vera gel significantly affected the pH and titratable acidity (p>0.05), but its effect on physical instability was not significant. The results suggest that due to the hydrophilic and anionic character of the hydrocolloids, they are useful in the processes of steric and electrostatic stability of TTB.
Keywords:
Introduction
In Colombia, the tree tomato (Cyphomandrabetacea) is a promising fruit for exportation and the orange variety is the most internationally accepted. Its pulp is very appreciated for its pleasant aroma, bittersweet-astringent flavor and orange color. It is a fruit with a high content of vitamins, minerals, carotenoids, anthocyanins and other phenolic compounds (Márquez et al., 2007). These compounds are not only responsible for the color of the fruit, but also have biological, therapeutic and preventive properties (do Nascimento et al., 2013; Castro-Vargas et al., 2013). The pulp is used in the production of juices, nectars, jams, salads and preserves (Márquez et al., 2007).
The fruit pulp is composed ofa significant amount of insoluble polymer material, responsible for increasing colloidal instability leading to the separation of phases in food suspensions during storage (Genovese et al., 1997). The phenomenon of separation phases in food products, due to the sedimentation of dense particles or agglomeration of particles associated with electrostatic attraction, is a quality defect that affects the stage of commercialization and acceptability of the product (Ibrahim et al., 2011).
To avoid the separation of phases in food suspensions, it has opted for the inclusion of hydrocolloids, substances of anionic character that canincrease the forces of electrostatic repulsion between particles. The Xanthan Gum (XG) and carboxymethylcellulose (CMC) are widely used hydrocolloids due to their high-water retention capacity and regulation of rheological characteristics increasing the viscosity of the suspension, creating a gel-like structure and providing steric and electrostatic stability of insoluble particles (Genovese and Lozano, 2001). Furthermore, several investigations related to the use of hydrocolloids (such as XG, CMC, guar gum, gellan, pectin, chitosan, among others) in food dispersions have reported essential changes in the rheology and colloidal stability of suspensions without affecting their commercial quality (Genovese and Lozano, 2001; Sahin and Ozdemir, 2007; Ghafoor et al., 2008; Ibrahim et al., 2011; Abbasi and Mohammadi, 2013; Lins et al., 2014; Nwaokoro and Akanbi, 2015).
Additionally, several studies highlight the functional properties of aloe vera (Aloe barbadensis Miller), mentioning that the inclusion of these components in food strengthens its nutraceutical value. However, aloe vera gel is a mucilaginous product that contains polymeric materials such as amino acids, lipids, minerals and vitamins; whose components in suspension affect the stability of dispersions (Habeeb et al., 2007; Boghani et al., 2012). In this context, the present work seeks to evaluate the effect of the addition of hydrocolloids and aloe vera on the physicochemical properties and its incidence in the degree of stability of tree tomato beverages.
Materials And Methods
Materials: Fruits of tree tomato (Cyphomandrabetacea) of the Sendt variety were used as the raw material. Additionally, sucrose as a sweetener, stabilizers such as Xanthan Gum (XG) and Carboxymethylcellulose (CMC) and aloe vera gel with 98% purity and food grade preservatives were used. These additives were supplied by the company Bell Chem International S.A. (Medellín, Colombia).
Formulation of the beverages: The formulation was based on the Colombian Technical Standard (NTC 3549/1999), which states that TTBs must have a minimum pulp content of 18% and a concentration of Total Soluble Solids (TSS) of 10°Brix. The respective amount of hydrocolloids and aloe vera was added before preparation in a water suspension at 40°C. A mixture of preservatives to the 0.125% w/w were addedto a 50/50 ratio (potassium sorbate/sodium benzoate). TTBs was homogenized in a disperser (Ultra Turrax, IKA T25, Germany) for 60 s at 5000 rpm and subjected to a slight pasteurization process for one 1 min at 60°C.
Physicochemical properties: The TTBs were characterized quantifying the concentration of Total Soluble Solids (TSS), pH, titratable acidity expressed as a percentage of citric acid and density based on the AOAC standard (2005). The measurements were made in triplicate a temperature of 25°C.
Determination of zeta potential (ζ): This parameter was determined by electrophoresis using a nano-sizer (Model ZS90, Malvern, UK) based on the protocol cited by Genovese and Lozano (2001) with slight modifications. The samples were diluted in distilled water, under a 1:20 ratio. The study was carried out in triplicate at a temperature of 25°C.
Particle size distribution: The particle size distribution in TTBs was evaluated by light scattering using Mastersizer equipment (Model 3000E, Malvern, UK) guided in the methodology proposed by Kubo et al., (2013). The size of the particles was expressed as a function of the mean diameter based on the surface area ratio (D [3, 2]) and the volume ratio (D [4, 3]) according to the expressions Eq. (1) and (2). Where, ni is the number of particles with diameter di:
$ D[3,2] = \dfrac {\Sigma_i n_i d_i^3}{\Sigma_i n_i d_i^2} ~~~~~~~~~~~~~~~~~~~~~~~~ (1)$
$ D[4,3] = \dfrac {\Sigma_i n_i d_i^4}{\Sigma_i n_i d_i^3} ~~~~~~~~~~~~~~~~~~~~~~~~ (2)$
Both relations were studied since D [4,3] is influenced by large particles, while small particles more influenced diameter D[3,2].
Turbidity (Cloudiness): The beverages were subjected to an accelerated sedimentation process using a centrifuge (Hettich, 320R, Germany) at 4200 g for 15 min in 25 mL graduated tubes (Ghafoor et al., 2008). The supernatant was collected and the absorbance at 660 nm was determined in a UV-visible spectrophotometer (Thermo-Scientific, E-60S, USA) taking as standard distilled water.
Sedimentation test: Phase separation in TTBs was studied based on the volume change in graduated cylinders of 50 mL (Fasolin and da Cunha, 2012). The analyzes were carried out at a temperature of 25°C, for 120 h until the equilibrium of the phases was reached. The Sedimentation Index (SI) was calculated by the following expression Eq. (3):
$ SI(\text {%}) = \Big( \dfrac {H_t}{H_o} \Big) * 100 ~~~~~~~~~~~~~~~~~~~~~~~~ (3)$
where, Vt is the volume of the upper phase at the interface after a time t and Ho the initial volume. The effect on the sedimentation process was analyzed using the first-order kinetic Eq. (4):
$ SI = SI_{eq} \Big( 1-e^{-vt} \Big) ~~~~~~~~~~~~~~~~~~~~~~~~ (4)$
where,
$ \begin{aligned}
SI_{eq} & = \text { The volume of sediment in the equilibrium } \\
t & = \text { The time } \\
v & = \text { The sedimentation velocity in } \; h^{-1}
\end{aligned} $
Finally, the phases were separated and characterized individually, evaluating properties such as density, particle size, viscosity and ζ potential.
Color: The color measurement was carried out by the CIELAB tristimulus system (L, a*, b*) using a sphere spectrophotometer (Model CR400, Konica-Minolta). The luminosity parameter (L) ranges from 0 (black) to 100 (white), the parameter a* indicates the degree of the color red (+ a*) or green (-a*) and the parameter b* measures the degree of yellow (+b*) or blue (-b*). The results were expressedby reference to the illuminant D65 and with a visual angle of 10° (Martin-Diana et al., 2009). From the CIELAB color spectrum, the color difference (ΔE) was determined by the following expression Eq. (5):
$ ∆E^* = \sqrt {(∆L^* )^2+(∆a^* )^2+(∆b^* )^2} ~~~~~~~~~~~~~~~~~~~~~~~~ (5)$
Experimental design: A composite central rotational designwas established with axial points and four replicates at the center point. The established factors and levels in the study are defined in Table 1. The experimental data were statistically analyzed with a significance level of 5% with the response surface analysis, analysis of variance, lack-of-fit test and determination of regression coefficients using the Stat graphics Centurion XVI software (Version 16.1.18, USA). The experimental data were adjusted with the second-order model Eq. (6):
$ Y = \beta_O + \Sigma_{i=1}^3 \beta_i X_i + \Sigma_{i=1}^3 \beta_{ii} X^2_i + \Sigma\Sigma_{i \le j=1} ^3 \beta_{ij} X_i X_j ~~~~~~~~~~~~~~~~~~~~~~~~ (6)$
where, $\beta_O, \; \beta_i, \; \beta_j$ and $\beta_ij$ are the regression coefficients for the intercept, linear and quadratic interaction terms, respectively and X is an independent variable.
Treatments | Aloe vera (%) | XG (%) | CMC (%) | Aloe vera $(X_1)$ | XG $(X_2)$ | CMC $(X_3)$ |
---|---|---|---|---|---|---|
T1 | 1.0 | 0.050 | 0.092 | 1.68 | 0 | 0 |
T2 | 1.0 | 0.008 | 0.050 | 0 | 0 | -1.68 |
T3 | 0.16 | 0.050 | 0.050 | 0 | -1.68 | 0 |
T4 | 0.5 | 0.075 | 0.075 | 1 | -1 | 1 |
T5 | 1.5 | 0.025 | 0.075 | 1 | 1 | -1 |
T6 | 1.0 | 0.050 | 0.050 | 0 | 0 | 0 |
T7 | 1.5 | 0.075 | 0.025 | -1 | 1 | 1 |
T8 | 1.0 | 0.050 | 0.050 | 0 | 0 | 0 |
T9 | 1.5 | 0.025 | 0.025 | -1 | 1 | -1 |
T10 | 1.0 | 0.050 | 0.008 | -1.68 | 0 | 0 |
T11 | 1.0 | 0.092 | 0.050 | 0 | 0 | 1.68 |
T12 | 1.84 | 0.050 | 0.050 | 0 | 1.68 | 0 |
T13 | 1.0 | 0.050 | 0.050 | 0 | 0 | 0 |
T14 | 1.0 | 0.050 | 0.050 | 0 | 0 | 0 |
T15 | 0.5 | 0.025 | 0.075 | 1 | -1 | -1 |
T16 | 1.5 | 0.075 | 0.075 | 1 | 1 | 1 |
T17 | 0.5 | 0.025 | 0.025 | -1 | -1 | -1 |
T18 | 0.5 | 0.075 | 0.025 | -1 | -1 | 1 |
Control | - | - | - | - | - | - |
* T17 (Minimum point); T6, T8, T13, T14 (Central points); T16 (Maximum point) |
Results And Discussion
Physicochemical properties: The addition of XG and CMC did not show a significant effect (p>0.05) on the behavior of physicochemical properties in TTBs. Similar results were reported in clarified fruit juices after the inclusion of chitosan (Chatterjee et al., 2004). Likewise, Chaikham and Apichartsrangkoon (2012) report that XG did not influence the assessment of the pH and titratable acidity of Dimocarpuslongan juices. However, the inclusion of aloe vera affected the pH and acidityof the TTBs formulated, as shown in Fig. 1, where was observed a significant increase (p<0.05) in the percentage of acidity correlated with a decrease in pH. The above is probably due to the presence of organic acids (such acitric, malic and acetic acid) constituents of the aloe vera gel intensifying the acidity in the TTBs (Boghani et al., 2012).
The pH value in the TTBs fluctuated between 4.090-4.281, values lower than the estimate in the TTB control (Table 2). Likewise, there was an increase in the percentage of acidity in the formulated TTBs concerning the TTB control, possibly associated with the inclusion of the aloe vera. Several authors reported a significant increase in titratable acidity and a change in pH of mango nectars formulated with aloe vera (Elbandy et al., 2014). However, with the addition of aloe vera gel in TTBs, the concentration of SST and density was not affected (Table 2). The above is possibly due to the low contribution of solids and high-water content that the gel possesses, what mildly affect the physicochemical behavior of the TTBs. Similar results were reported in mango and tangerine nectars, enriched with aloe vera gel between 4 to 10% (Elbandy et al., 2014; Shubhra et al., 2014).
On the other hand, the regression models presented a coefficient of determination (R2) of 0.801, 0.774, 0.824 and 0.675 for the behavior of physicochemical properties as pH, acidity, SST and density in the respective order (Table 3) with lack-of-fit test no statistically significant (p>0.05).
Zeta potential (ζ): A significant effect was determined (p<0.05) on the inclusion of XG in the ζ potential behavior in TTBs (Fig. 2). This behavior was probably associated to the anionic character of the XG and its ability to induce highly repulsive forces, preventing the colloidal particles from uniting and provoke rupture mechanisms, affecting the stability of suspensions (Mirhosseini and Tan, 2010). Similar results were reported in the colloidal stability of apple juice formulated with XG (Genovese and Lozano, 2001). Dluzewska et al. (2005) argue that hydrocolloids can stabilize dispersions through viscous effects, steric or electrostatic interactions.
The values of ζ potential in the formulated TTBs ranged from -27.567 to -42.667 mV (Table 4), similar results to those reported in apple juices, stabilized with XG and CMC (Genovese and Lozano, 2001). Likewise, ζ potential values were reported between -24.6 and -39.1 in orange beverages, formulated with pectin and CMC (Dluzewska et al., 2005). Authors mention that a ζ potential differential more significant than 25 mV is indicative of stable emulsion systems without agglomeration effect (Leiberman et al., 1998; Schramm, 2005). In turn, Abbasi and Mohammadi (2013) highlight the stabilizing power of guar gum in milk emulsions and orange juice, from the ζ potential values that fluctuated from -24 to -31 mV. Consequently, the stabilizing capacity of the XG and CMC in the TTBs is obtained, with respect to the control treatment (-18.16 mV).
Particle size: The results show values more significant of the diameter D [4,3] (influenced by large particles) concerning the diameter D [3,2] (affected by smaller particles), it is explaining the differences in size particles associated to the quantification method (Table 4). The addition of hydrocolloids and aloe vera, they did not show a significant effect on the variation of the mean diameter of the suspensions (p>0.05). However, it was determined a slight increase in the diameters D [3,2] andD [4,3] of the TTBs treated concerning the control. Studies have reported an increase in the average particle diameter after the addition of hydrocolloids in food dispersions (Huang et al., 2001). Moreover, is declareda rise between 2 to 20 μm in the diameter D [4,3] in carrot pulp particles due to the addition of gellan gum (Sherafati et al., 2013). In turn, authors report that the addition of polymeric materials as aloe vera gel in suspensions can produce variation in the particle size, due to the presence of polysaccharide substances with encapsulating capacity (Keshtkaran et al., 2013).
On the other hand, the uniformity in the particle size distribution in the TTBs formulated with hydrocolloids possibly is due to the applied homogenization process. Silva et al. (2010) argue that the process of homogenization implies the reduction of particles, with to create a stable dispersion. The regression models that describe the behavior of the diameters D [3,2] and D [3,4] presented coefficients R2 of 0.812 and 0.744, respectively (Table 3) with a lack-of-fit test not statistically significant (p>0.05). The mean diameters D [3,2] and D [4,3] in the treated TTBs ranged between 136-150 μm and 235-265 μm, respectively. These values are similar to those reported intomato and orange juices (Kubo et al., 2013; Leite et al., 2014).
Turbidity (Cloudiness): The incorporation of XG, turned out to be significant (p<0.05) on the behavior of the turbidity or cloudiness of TTBs. The presence of hydrocolloids increased the turbidity in the supernatant, indicating greater retention of particles in suspension and a decrease in the phenomenon of phase separation (Fig. 2). Some authors consider that the turbidity in suspensions is the result of insoluble particles dispersed in the continuous phase (Silva et al., 2010). Similar behavior was reported in grape juices stabilized with XG, gellan and carrageenan (Ghafoor et al., 2008).
It was observed a significant increase in turbidity with the gradual increase in the concentration of XG. Mirhosseini and Tan (2010) report an increase in the turbidity of orange emulsions stabilized with pectin and CMC. In turn, Genovese and Lozano (2001) found an improvement in the stability of apple juices through the incorporation of CMC and XG, associated with the increase of the forces of electrostatic repulsion between particles product of a stabilizing effect in the suspensions and greater turbidity.
After the accelerated sedimentation process by centrifugation was achieved the separation into two phases of the TTBs. However, a direct relationship was observed between the ζ potential values, turbidity and sedimentation index in the TTBs formulated with concentrations higher than 0.05% of XG (T4, T11, T16), these factors are indicators of excellent stability of particles in suspension. Some authors managed to establish similar relationships during the stability study of fruit juices stabilized with hydrophilic colloids (Benítez and Lozano, 2007).
Sedimentation velocity: Table 4 details the parameters that describe the phenomenon of phase separation in the TTB during storage, as a function of the index (SIeq) and sedimentation rate (v). The SI decreases significantly with the increase in hydrocolloid concentration (Table 4), results similar to those reported in tomato-carrot mixture juices stabilized with CMC, XG and guar gum (Nwaokoro and Akanbi, 2015). On the other hand, in those treatments where CMC and XG were incorporated at high concentrations (>0.05%), no sedimentation phenomena were detected during storage (SI = 0). This behavior could be due to the synergistic effect of the stabilizers, where the XG has a greater capacity to increase the apparent viscosity, while the CMC gum by its electronegativity, increases the electrostatic repulsion forces between particles, providing greater stability to TTBs (Genovese and Lozano, 2001). A similar behavior is reported in grape juices highlighting the stabilizing power of XG, gellan and carrageenan controlling phenomena of colloidal instability such as precipitation or agglomeration of particles (Ghafoor et al., 2008).
The addition of XG show a significant effect on the decrease of the sedimentation velocity (Fig. 2), reducing the phase separation in TTBs, results consistent with the increase of the electrostatic differential between particles measured through the ζ potential. Ghafoor et al. (2008) argue that XG is an anionic polysaccharide with the ability to increase electrostatic repulsion between particles, maintaining its colloidal state in suspension (Genovese and Lozano, 2001; Moraes et al., 2011). Likewise, XG has a high molecular weight that favors the increase of the apparent viscosity of the continuous phase, decreasing particle precipitation (Sahin and Ozdemir, 2007). Stokes' law illustrates that the sedimentation rate of suspended particles is inversely proportional to their viscosity, which means that a higher viscosity can beneficially increase steric stability in fruit juices (Chaikham and Apichartsrangkoon, 2012; Paquet et al., 2014; Cho and Yoo, 2015). Besides, Sahin and Ozdemir (2007) found that the XG presented an excellent behavior as a stabilizer of tomato sauces, associated with its remarkable water retention capacity affecting the rheology of the formulated suspensions.
The sedimentation velocity ranged between 0-0.130 h-1 (Table 4), these values are higher than those reported in soursop juice stabilized with soy protein (Fasolin and da Cunha, 2012). In turn, the regression model that describes the behavior of sedimentation velocity in function of the addition of hydrocolloids and aloe vera presented a high coefficient of determination of 0.936 and the lack-of-fit test no statistically significant (p>0.05).
Phase characterization: The characterization of the phases in TTBs was carried out to understand the phenomenon of instability. It was not detected significant differences in density behavior, the ζ potential difference and average particle diameters about the concentration of hydrocolloids and aloe vera (p>0.05). Both the density and the particle size of the sedimented phase are higher than those estimated in the supernatant phase (Table 5), these differences can be considered as precursor factors of instability mechanisms in the TTBs. Schramm (2005) expresses that a reduction in particle size leads to slow phase separation by gravitational effect since based on Stokes' law the sedimentation velocity is proportional to the square of the diameter of the particles. Also, smaller particles have less weight-lower density-precipitating less. Thus, McClements (2005) argues that large particles tend to have a higher tendency to coalesce than small particles, therefore, reducing the size can increase the stability and extend the useful life of colloidal dispersions. On the other hand, the average diameters in TTBs formulated with hydrocolloids concerning the control, did not present significant differences possibly associated with the applied homogenization process.
The viscosity of the supernatant phase increased significantly as a function of the addition of XG and CMC (p<0.05), highlighting the preponderant effect of the XG due to its high water retention capacity (Fig. 2). In turn, the increase in viscosity in the supernatant phase corresponds to a more significant presence of particles in suspension and greater electrostatic potential indicators of stabilizing effects in the TTBs. About the ζ potential differential in the supernatant phase, there is a significant increase in the treated TTBs compared to the control, associated with the presence of anionic substances. Consequently, it can be inferred that the physical stability of the TTBs with the addition of hydrocolloids are related to steric processes (increase in viscosity of the continuous phase) and electrostatic processes (a growth of repulsive forces between particles).
The regression model that simulates the behavior of the viscosity in the supernatant phase was high (R2 = 0.90) and the lack-of-fit test was not statistically significant. Meanwhile, the sedimented phase viscosity did not show a considerable behavior in function of the concentration of hydrocolloids and aloe vera (p>0.05). However, the viscosity was lower than that estimated in the control sample; it trends that may be associated with the reduction of phase separation or particle sedimentation processes. In turn, the sedimented phase in the treated TTBs has a more significantζ potential differential possibly due to the presence of the anionic substance.
Instrumental color determination: The addition of hydrocolloids and aloe vera did not significantly influence (p>0.05) the variation of the color parameters of the TTBs (Table 2). The luminosity parameter (L) in the TTBs increased with the incorporation of hydrocolloids concerning the control, a result similar to that reported in enriched orange juices stabilized with chitosan (Martin-Diana et al., 2009). Authors suggest that L tends to decrease in beverages o suspensions attributed to the decantation of particles in time (Genovese et al., 1997). However, the values of L in the TTBs treated with XG and CMC did not show this tendency, possibly due to the increase in clarity of the suspension associated with the use of hydrophilic substances essentially at high concentrations (Chatterjee et al., 2004; Halim et al., 2014). The values of L in the treated TTBs oscillated between 36.470 and 43.657 higher than those estimated in the control drink (L = 39.047) differences explained by the addition of XG and CMC.
The positive values of a* oscillated between 3.023 and 4.868 indicating a visual position regarding the red color, while the positive values of b* varied between 9.800 and 12.807 showing a tendency toward yellow. It observed a decrease in the color parameters a* and b* with the incorporation of XG and CMC, compared to the control, results according to those cited in fermented cassava ice cream formulated with XG and CMC (Halim et al., 2014). The regression models that explain the behavior of the parameters L, a* and b* in function of the concentration of hydrocolloids and aloe vera gel showed R2 coefficients of 0.685, 0.613 and 0.751, respectively (Table 3).
The variation of the color (ΔE) in the TTBs ranged from 0.732 to 2.402 warning a significant decrease in the TTBs treated concerning the control beverage (Table 2). Nwaokoro and Akanbi (2015) explain that retention of the color in fruit juices is possibly due to the ability of anionic biopolymers to encapsulate particles in suspension and control the phenomena of precipitation and oxidative degradation of pigments. Similar behavior was evaluated with the addition of pectin and gelatin that contributed to the control of color loss in restructured fruits (Lins et al., 2014). In turn, Martin-Diana et al. (2009) indicate that changes in color parameters are strictly related to chitosan treatments in orange juice. The regression model representing the behavior of the color change showed a coefficient of determination of 0.754 and the lack-of-fit test was not significant (Table 3).
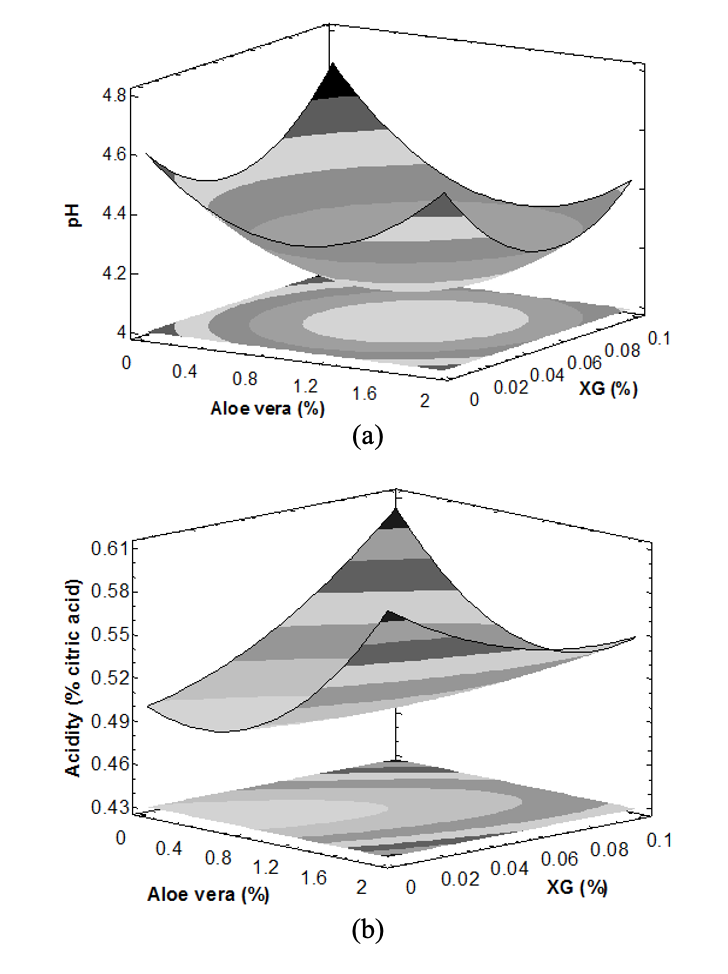
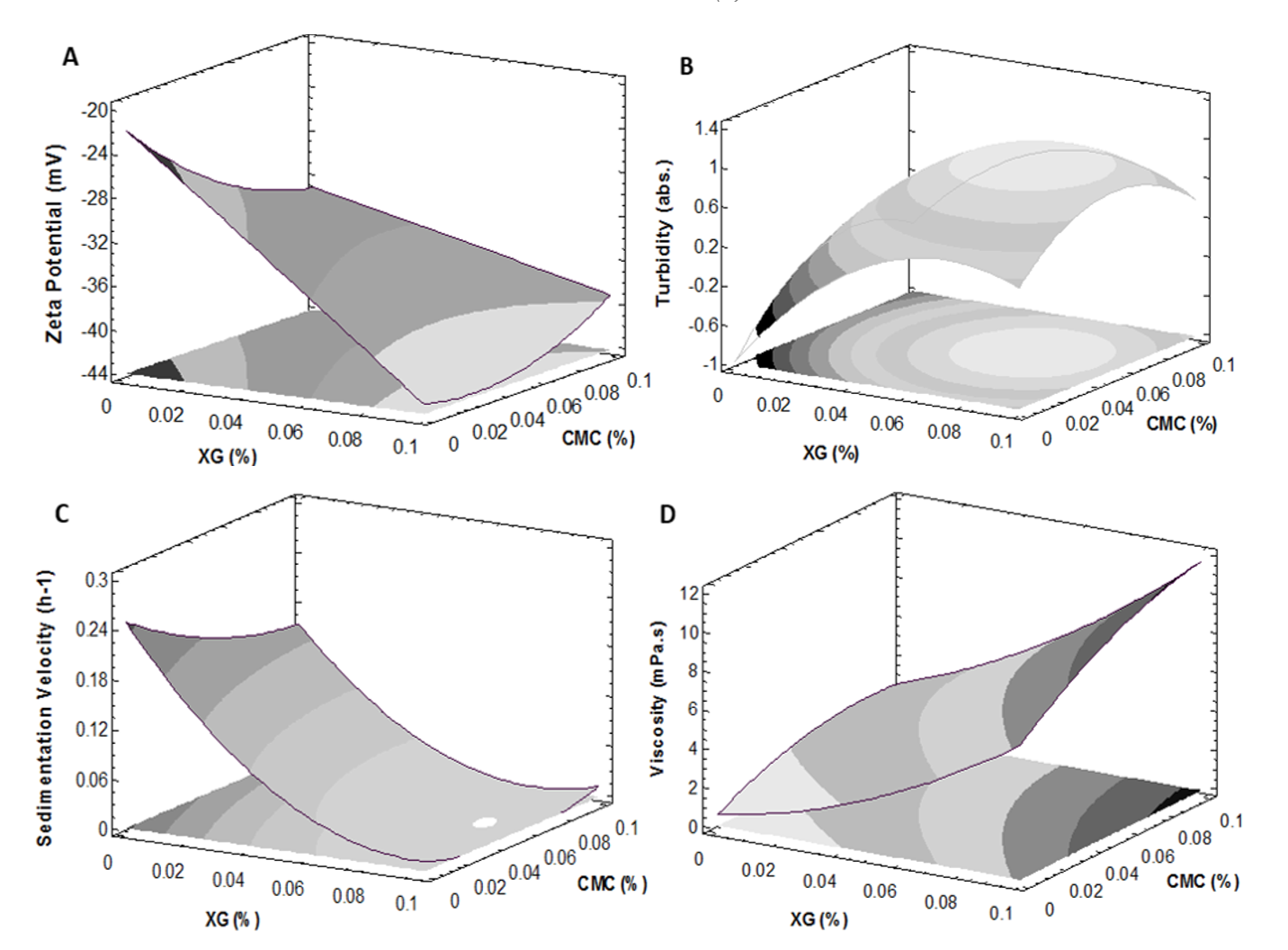
Treatments | pH | Acidity (% citric acid) | Density (g/mL) | TSS (°Brix) | L | a* | b* | ΔE* |
---|---|---|---|---|---|---|---|---|
T1 | 4.281 | 0.495 | 1.0310 | 9.567 | 39.040 | 4.503 | 12.463 | 0.958 |
T2 | 4.310 | 0.512 | 1.0290 | 9.367 | 39.117 | 4.123 | 11.057 | 2.041 |
T3 | 4.346 | 0.530 | 1.0250 | 9.800 | 39.003 | 3.733 | 10.627 | 1.045 |
T4 | 4.337 | 0.512 | 1.0260 | 9.833 | 39.030 | 3.897 | 10.530 | 0.857 |
T5 | 4.247 | 0.524 | 1.0090 | 9.467 | 40.837 | 4.120 | 10.830 | 1.124 |
T6 | 4.190 | 0.499 | 0.9910 | 9.333 | 38.273 | 4.177 | 10.637 | 0.920 |
T7 | 4.011 | 0.515 | 1.0210 | 9.400 | 39.760 | 3.313 | 09.923 | 0.803 |
T8 | 4.097 | 0.495 | 0.9880 | 9.333 | 38.195 | 4.668 | 10.615 | 1.258 |
T9 | 4.096 | 0.522 | 1.0070 | 9.367 | 38.860 | 4.060 | 10.680 | 1.941 |
T10 | 4.185 | 0.500 | 1.0240 | 9.667 | 40.277 | 3.987 | 12.407 | 1.061 |
T11 | 4.308 | 0.518 | 1.0220 | 9.700 | 38.963 | 3.560 | 10.093 | 0.924 |
T12 | 4.310 | 0.539 | 1.0270 | 9.700 | 38.827 | 3.577 | 10.160 | 1.647 |
T13 | 4.093 | 0.506 | 1.0210 | 9.533 | 39.666 | 3.818 | 09.980 | 1.479 |
T14 | 4.090 | 0.509 | 0.9770 | 9.400 | 36.470 | 4.198 | 10.690 | 0.921 |
T15 | 4.256 | 0.435 | 1.0290 | 9.600 | 41.987 | 3.210 | 10.017 | 2.402 |
T16 | 4.207 | 0.532 | 1.0240 | 9.633 | 42.850 | 3.023 | 09.800 | 0.732 |
T17 | 4.321 | 0.540 | 0.9770 | 9.567 | 43.657 | 3.970 | 12.480 | 1.419 |
T18 | 4.246 | 0.542 | 1.0330 | 9.433 | 40.553 | 3.087 | 10.077 | 0.836 |
Control | 4.350 | 0.485 | 1.0466 | 9.900 | 37.675 | 4.799 | 12.560 | - |
Coefficients regression | TSS (°Brix) | Density (g/mL) | D [3, 2] (µm) | D [4, 3] (µm) | L | a* | b* | ΔE |
---|---|---|---|---|---|---|---|---|
$\beta_0$ | 9.402 | 0.994 | 143.196 | 240.605 | 38.067 | 4.201 | 10.387 | 1.088 |
$\beta_1$ | -0.083 | 0.003 | 0.366 | -7.06 | -0.235 | 0.006 | -0.194 | 0.007 |
$\beta_2$ | 0.092 | 0.002 | 1.128 | 0.041 | -0.249 | -0.218 | -0.388 | -0.406 |
$\beta_3$ | 0.014 | 0.005 | 1.152 | -2.832 | -0.015 | 0.050 | -0.188 | -0.004 |
$\beta_{11}$ | 0.110 | 0.010 | NS | 3.975 | 0.634 | -0.267 | -0.159 | 0.077 |
$\beta_{12}$ | -0.037 | -0.008 | NS | 2.500 | 1.122 | -0.206 | 0.013 | 0.075 |
$\beta_{13}$ | 0.037 | -0.005 | -2.625 | 3.750 | 1.033 | NS | 0.255 | -0.237 |
$\beta_{22}$ | 0.034 | 0.009 | 1.132 | 3.443 | 0.678 | -0.201 | NS | 0.125 |
$\beta_{23}$ | 0.012 | -0.007 | -1.375 | 3.000 | NS | 0.153 | 0.330 | NS |
$\beta_{33}$ | 0.063 | 0.010 | -1.171 | 2.558 | 0.897 | NS | 0.635 | NS |
$R^2$ | 0.823 | 0.675 | 0.812 | 0.744 | 0.685 | 0.613 | 0.751 | 0.754 |
Model (p-value) | 0.034 | 0.002 | 0.042 | 0.026 | 0.031 | 0.045 | 0.007 | 0.019 |
Lack-of-fit (p-value) | 0.417 | 0.756 | 0.752 | 0.242 | 0.516 | 0.667 | 0.113 | 0.419 |
Treatments | D [3,2] (µm) | D [4,3] (µm) | ζ Potential (mV) | Viscosity (mPa.s) | SI (%) | v (h-1) | R2 |
---|---|---|---|---|---|---|---|
T1 | 143 | 250 | -36.900 | 5.596 | 0.00 | 0.000 | NR |
T2 | 145 | 243 | -33.200 | 2.250 | 40.9 | 0.127 | 0.981 |
T3 | 145 | 265 | -37.400 | 4.946 | 4.50 | 0.027 | 0.926 |
T4 | 148 | 246 | -35.933 | 8.343 | 0.00 | 0.000 | NR |
T5 | 142 | 240 | -29.333 | 4.546 | 37.10 | 0.103 | 0.912 |
T6 | 142 | 237 | -38.267 | 5.545 | 4.06 | 0.024 | 0.898 |
T7 | 147 | 241 | -38.900 | 6.095 | 0.00 | 0.000 | NR |
T8 | 140 | 243 | -36.456 | 5.396 | 5.00 | 0.022 | 0.917 |
T9 | 142 | 247 | -30.533 | 2.698 | 39.5 | 0.113 | 0.988 |
T10 | 139 | 248 | -34.233 | 3.397 | 7.38 | 0.064 | 0.945 |
T11 | 150 | 260 | -41.000 | 9.242 | 0.00 | 0.000 | NR |
T12 | 145 | 241 | -36.200 | 4.946 | 24.5 | 0.028 | 0.949 |
T13 | 144 | 235 | -34.934 | 5.396 | 6.40 | 0.039 | 0.985 |
T14 | 145 | 247 | -35.123 | 5.045 | 3.50 | 0.022 | 0.907 |
T15 | 146 | 250 | -36.233 | 4.646 | 22.3 | 0.093 | 0.901 |
T16 | 148 | 242 | -42.667 | 8.742 | 0.00 | 0.000 | NR |
T17 | 136 | 256 | -27.567 | 2.947 | 55.7 | 0.130 | 0.939 |
T18 | 140 | 256 | -39.800 | 6.095 | 0.00 | 0.000 | NR |
Control | 139 | 231 | -18.167 | 4.500 | 67.50 | 1.541 | 0.946 |
Control beverage | Treated beverages | |||
---|---|---|---|---|
Physicochemical properties | Supernatant | Sediment | Supernatant* | Sediment* |
Density (g/mL) | 0.99±0.01 | 1.49±0.12 1.08±0.02 1.49±0.07 | ||
ζ potential (mV) | -19.13±1.83 | -31.37±2.21 | -34.14±2.18 | -42.92±3.68 |
D (3,2), µm | 7.08±1.43 | 151±3.53 | 9.08±1.44 | 157.61±4.12 |
D (4,3), µm | 29.60±2.81 | 249.56±12.32 | 35.88±3.15 | 271±12.84 |
Viscosity (mPa.s) | 4.50±0.37 | 3229.8±172 | -- | 2722.60±110 |
*Arithmetic mean±standard error; *Average values for the 18 tests |
Conclusion
The study has shown the significant effect of hydrocolloids on the degree of stability in Tree Tomato Beverages (TTBs). The incorporation of xanthan gum was significant in the decrease of the sedimentation velocity and increase of the electrostatic repulsion of particles (ζ potential >30 mV). Aloe vera gel intensified the pH and acidity but did not significantly influence (p>0.05) the degree of stability of the TTBs.
The addition of hydrocolloids increased the particle size, significantly affected the degree of stability, but did not influence the color variation of the TTBs. The results suggest that due to the hydrophilic and anionic nature of the hydrocolloids, the concentrations of xanthan gum and carboxymethylcellulose at 0.05% can be considered as the appropriate treatments in the colloidal stability of TTBs, without affecting the physicochemical properties.
Acknowledgement
The authors express their gratitude to the Research Direction of the Universidad Nacional de Colombia-Sede Medellin for economically funding this research.
Conflict of Interest
There is no “Conflict of interest” of the authors, with the results of the research work.
Author Details
1Facultad de Ciencias, Universidad Nacional de Colombia, Medellín, Colombia
2Facultad de Ciencias Agrarias, Universidad Nacional de Colombia, Medellín, Colombia
References
Rights and permissions
Open Access: This article is licensed under a Creative Commons Attribution 4.0 International License, which permits use, sharing, adaptation, distribution and reproduction in any medium or format, as long as you give appropriate credit to the original author(s) and the source, provide a link to the Creative Commons license, and indicate if changes were made. The images or other third-party material in this article are included in the article’s Creative Commons license, unless indicated otherwise in a credit line to the material. If material is not included in the article’s Creative Commons license and your intended use is not permitted by statutory regulation or exceeds the permitted use, you will need to obtain permission directly from the copyright holder. To view a copy of this license, visit http://creativecommons.org/licenses/by/4.0/
Cite this Article
DOI: http://doi.org/10.19026/ajfst.17.6009
Sections