Advance Journal of Food Science and Technology
Production of Functional Coenzyme Q10 from Genetic Engineered Rhodobactersphaeroides
Advance Journal of Food Science and Technology 2019 17: 48-53
Cite This ArticleAbstract
The aim of this study was to enhance the Coenzyme Q10 (CoQ10) production from Rhodobactersphaeroides. CoQ10 acts as an electron carrier and plays an important role in the aerobic respiration for the production of ATP. CoQ10 has been proven to be effective for treatment of human diseases and widely used in food and pharmaceutical industry. In the present study, the ubiE gene located in CoQ10 biosynthesis pathway was overexpressed in Rb. sphaeroides under strong pufoperon promoter and micro-aerobic growth conditions to enhance CoQ10 production. The growth curve implied that effect of overexpression of ubiE on the growth of host cells was slight. The crude CoQ10 production was enhanced by 80.61%, which was increased much higher than reported literature. The ubiE mRNA level was significantly increased compared to the wild type harboring empty vector as measured by quantitative RT-PCR. Furthermore, the crude CoQ10 possessed strong hydroxyl radical scavenging activity as measured in vivo by zone of inhibition assay. The in vitro assay indicated that the crude CoQ10 exhibited much stronger hydroxyl radical scavenging activity than normally used antioxidant vitamin C.
Keywords:
Introduction
CoQ10 (2, 3-dimethoxyl, 5-methyl, 6-decaisoprene parabenzoquinone), also referred as to ubiquinone 10, is a lipid-soluble material wide-spread in the plasma membrane of prokaryotes and inner mitochondrial membrane of eukaryotes. It has been proposed that CoQ10 has multiple functions, including transferring electron in the electron transport chain of aerobic respiration (Garrido-Maraver et al., 2014; Lenaz et al., 2007). Furthermore, CoQ10 is a well-known anti-oxidant since it can scavenge free radicals, which are generally harmful to phosphate lips, proteins and DNA (Cluis et al., 2007; Rizvi et al., 2015). CoQ10has been used to treat and prevent many diseases, including Parkinson’s disease, Alzheimer’s disease and mitochondrial diseases (Cluis et al., 2007; Hargreaves, 2014; Negida et al., 2016). CoQ10 becomes a good additive for functional foods because of its excellent anti-oxidant activity (Lee et al., 2013). However, external source of CoQ10 is required because dietary uptake for CoQ10 will be limited. Furthermore, endogenous synthesis in the body will be decreased with the age and under various diseases. With the applications of CoQ10 in many fields, demands for the CoQ10 are growing very rapidly and solutions for enhancing CoQ10 production have being tried by researchers.
Currently, CoQ10 is mainly produced by three approaches, chemical synthesis, semi-chemical synthesis and microbial fermentation. Among which, the microbial fermentation approach is becoming more and more popular due to the advantages that the bio-produced CoQ10 compared to chemical synthesis. It is thus that the microbial fermentation is attracting more and more attentions from researchers. At the beginning, much attention has been paid to the construction of high-yield microbial producer by metabolic engineering E. coli strains. However, genetic engineered E. coli strains could not enhance the production of CoQ10 efficiently because of low fermentation titer and emergence of byproduct like CoQ8 and CoQ9 (Cluis et al., 2012; Park et al., 2005).
Among all the microbial producers employed for natural CoQ10 production, Rb. sphaeroides is one of the most promising microorganisms (Zahiri et al., 2006; Zhu et al., 2017). The whole genome of Rb. sphaeroides has been completely sequenced, which greatly benefits the researches on the enzyme encoding genes involved in the CoQ10 biosynthesis pathway. Biosynthesis of CoQ10 in Rb. sphaeroides generally includes three pathways, the 2-C-Methyl-D-Erythritol 4-Phosphate (MEP) pathway, the shikimate pathway and the Quinine Modification Pathway (QMP). Researches have been intensively performed and progress on improving CoQ10 production in Rb. sphaeroides has been made in the past several years. However, the performed researches were mainly focused on optimization of fermentation procedures. UbiE is a methyltransferase, participating in catalyzing 2-Decaprenyl-6-methoxyphenol into 2-Decaprenyl-3-methyl-5-hydroxy-6-methoxy-1, 4-benzoquinone (Lu et al., 2015).
On the other hand, Rb. sphaeroides has been an excellent model for studying both photosynthesis and membrane development (Kiley and Kaplan, 1987). The pufoperon and pucoperon encodes light-harvesting complexes 1 (LH1), Reaction Center (RC) and LH2, respectively (Hu et al., 2002). Both puf and puc promoters are strong, which are regulated by oxygen tension (Hu et al., 2010). A strong promoter and optimal growth conditions are very important for the production of CoQ10 in genetic engineered Rb. sphaeroides.
Currently, enhancement of CoQ10 in Rb. sphaeroides by overexpression of ubiE under strong pufoperon promoter and micro-aerobic growth condition has not been reported. In the present study, we overexpressed the ubiE in Rb. sphaeroides under strong pufoperon promoter at micro-aerobic growth condition. The production of CoQ10 from the genetic engineered strain was enhanced by 80.61%, which was increased much higher than reported literature (Lu et al., 2015). Our study will promote the application of Rb. sphaeroides for large scale production of functional CoQ10.
Materials And Methods
Bacterial strains and growth conditions: Rb. sphaeroides strains were grown at 30°C in malate minimal medium (Remes et al., 2014). Growth under micro-aerobic conditions was performed as described in our previous study (Hu et al., 2010). E. coli strains were grown aerobically at 37°C in Luria-Bertani medium. Antibiotics were added to the growth media at the following concentrations when necessary: 200 μg/mL ampicillin, 20 μg/mL tetracycline for E. coli and 1.5 μg/mL tetracycline for Rb. sphaeroides.
Construction of expression vector: The ubiE was first amplified from Rb. sphaeroides genomic DNA by PrimeSTAR HS DNA polymerase (TAKARA) with the primers of ubiE-F (5’-CGGGATCCATGAGCGACGA AACTTCC-3’) and ubiE-R (5’-GGGGTACCTCAGAT CTTCCAGCCGG-3’) and ligated into cloning vector pMD18-T (TAKARA). After sequence, the pMD18-ubiE plasmid was digested by KpnI and BamHI and the ubiE fragment was subsequently purified by gel extraction and ligated into pRKpuf (Hendrischk et al., 2009) digested by KpnI-BamHI, resulting in the pRK ubiE overexpression vector.
Construction of the genetic engineered strain: The constructed plasmid DNA was mobilized into Rb. sphaeroides 2.4.1 by using E. coli S17-1 as the donor as described in our previous study (Hu et al., 2010).
Determination of the high yield CoQ10 genetic engineered strain: After conjugation, three colonies of the conjugant were selected and cultured respectively in three 50 mL-flasks containing 40 mL of malate minimal media with 1.5 µg/mL tetracycline and grown under micro-aerobic conditions in the dark at 30°C until OD660 reached approximately 0.6. Pre-cultures were respectively inoculated into three 100-mL flasks containing 80 mL of malate minimal media with 1.5 µg/mL tetracycline at the ratio of 1% and grown under micro-aerobic conditions in the dark at 30°C for 48 h. Crude CoQ10 was extracted from the cell cultures and quantified as described by Chen et al. (2006), respectively.
Quantitative RT-PCR: Total RNA for qRT-PCR was isolated from cell cultures using the Tiangen Bacteria RNA Isolation Kit (#DPN430) as described by the manufacturer. mRNA from genetic engineered strain and wild type strain harboring empty vector was considered sample mRNA and control mRNA, respectively. To further confirm the absence of DNA, PCR was performed targeting gloB (RSP_0799). qRT-PCR was performed as described previously (Remes et al., 2014) in a Bio-Rad CFX96 Real Time system by using the primers, ubiE-real-F (5’-GTTCCGCTTCCTCAAGCG-3’) and ubiE-real-R (5’-GACGTCGAACGTGTTCGA G-3’). Relative mRNA expression levels were normalized to the reference gene rpoZ (Zeller et al., 2007) according to the formula given by Pfaffl (2001).
Hydroxyl radical scavenging activity: Hydroxyl radical was generated by the Fenton system (Sachindra et al., 2007). The A0 reaction mixture contained 1, 10-Phenanthroline monohydrate (1 mL, 0.75 mM), PBS solution (3 mL, pH7.4, 0.2 M), H2O (1 mL), FeSO4 (1 mL, 0.75 mM), H2O2 (1 mL, 0.01%). The A1 reaction mixture was the same as A0 reaction mixture without the H2O2, which was replaced by H2O. However, the A2 reaction mixture was the same as A0 reaction mixture without 1 mL of H2O, which was replaced by various concentrations of crude CoQ10 or vitamin C. All the reaction mixtures were incubated at 37°C for 60 min. The OD was measured at 510 nm and the results were used to calculated the scavenging percent as follows:
Zone of inhibition: Zone of inhibition assay was performed as described in our previous study (Zhao et al., 2018). Filters soaked with 5 µL of 700 mM H2O2 were placed on the top of the plates.
Data analysis: All experiments were repeated three times. Turkey test and GraphPad Prism software were used to analyze the data trend.
Results And Discussion
Construction of the expression vector: The expression vector used in this study was constructed as shown in Fig. 1. The UbiE is an important catalyzed enzyme in the ubiquinone pathway for the biosynthesis of CoQ10 in Rb. sphaeroides (Lu et al., 2015), which is a dimethyl menaquinone methyltransferase, involved in catalyzing 2-Decaprenl-6-methoxyphenol into 2-Decap-renyl-3-methyl-5-hydroxy-6-methoxy-1,4-benzoquione. pufoperon encodes the LH1 and reaction center in Rb. sphaeroides (Eisenhardt et al., 2018; Gong and Kaplan, 1996). The pufoperon promoter is a strong promoter responsible for two transcripts, a 2.7-kb transcript for pufBALMX and a 0.5-kb transcript for pufBA (Gong et al., 1994). Under the micro-aerobic growth conditions, the pufoperon promoter exhibits stronger activity. On the other hand, the pRK415 vector (Billenkamp et al., 2015) is a broad host range expression vector. Consequently, the ubiE will be efficiently expressed in Rb. sphaeroides under the strong pufoperon promoter grown at micro-aerobic growth conditions.
Construction of the genetic engineered strain for overexpression of UbiE: The constructed expression vector was mobilized into Rb. sphaeroides by conjugation via S17-1 as the donor. To test whether overexpression of UbiE affects the growth of the host cells, the growth curves for wild type 2.4.1, 2.4.1/pRKpuf and 2.4.1/pRKubiE were constructed as shown in Fig. 2. It was obvious that significant differences between the three different strains were not observed. Especially for the first 24 h, the growth curves were nearly overlapped, suggesting that the three different strains were grown at the same rate. In the following 12 h, the growth of all the strains nearly stayed the same tendency. It has been suggested that the CoQ10 plays indispensable roles not only in energy generation but also in many other processes that are important for cell’s survival (Zahiri et al., 2006). However, in the present case, production of CoQ10 probably did not affect the growth of the host cells.
It is reasonable to demonstrate that overexpression of ubiE gene do enhance the CoQ10 production in Rb. sphaeroides. Consequently, crude CoQ10 was extracted from the genetic engineered strain, as shown in Fig. 3. It was evident that production of crude CoQ10 from the genetic engineered strains 2.4.1/pRKubiE was increased a lot compared to the wild type strain 2.4.1 and 2.4.1/pRKpuf. The production of crude CoQ10 from the 2.4.1 and 2.4.1/pRKpuf was nearly the same, indicating that expression of the empty vector did not affect the production of crude CoQ10. Compared to the wild type, the CoQ10 production in 2.4.1/pRKubiE, was increased by 80.61%, which was much higher than the reported study (Lu et al., 2015). It can be suggested that overexpression of the ubiE lead to the obvious enhancement of the CoQ10 production under the strong pufoperon promoter and micro-aerobic growth conditions, suggesting the strong activation by the pufoperon promoter (Lu et al., 2015). On the other hand, the expression vector used in this study is a broad-host range vector to a wide range of Gram-negative bacteria.
Quantitative RT-PCR analysis for ubiE gene: To further test the reasons caused the obvious enhancement of CoQ10 from the genetic engineered strain 2.4.1/pRKubiE, qRT-PCR was employed, as shown in Fig. 4. As expected, the ubiE mRNA level was significantly increased, with log2 fold change of approximately 13. UbiE is a methyltransferase, participating in catalyzing 2-Decaprenyl-6-methoxyphenol into 2-Decaprenyl-3-methyl-5-hydroxy -6-methoxy-1, 4-benzoquinone (Lu et al., 2015). The UbiE expression level should be increased because of the increased ubiE mRNA expression levels and CoQ10 production.
Hydroxyl radical scavenging activity: From the in vivo experiment of zone of inhibition, it could be concluded that the strain 2.4.1/pRKubiE possessed much higher hydroxyl radical scavenging activity than that of the control strain 2.4.1/pRKpuf, as revealed in Fig. 5A. The H2O2 is a normally used oxidant produced ˖OH radical by Fenton reaction (Fischbacher et al., 2017). Obviously, the control strain 2.4.1/pRKpuf was more sensitive to H2O2 than that of the sample strain 2.4.1/pRKubiE, because the size of zone for 2.4.1/pRKpuf was bigger than that of 2.4.1/pRKubiE. On the other hand, CoQ10 exhibited much higher hydroxyl radical scavenging activity produced by H2O2 in vitro than that of the commonly used antioxidant vitamin C, since the IC50 for crude CoQ10 was much lower than that of vitamin C, as shown in Fig. 5B. It was surprised that the crude CoQ10 had so much higher hydroxyl radical scavenging activity, indicating the crude CoQ10 was functional and thus possessed the potential for commercial utility in food, pharmaceutical and cosmetic industries after further purification by HPLC or other techniques.
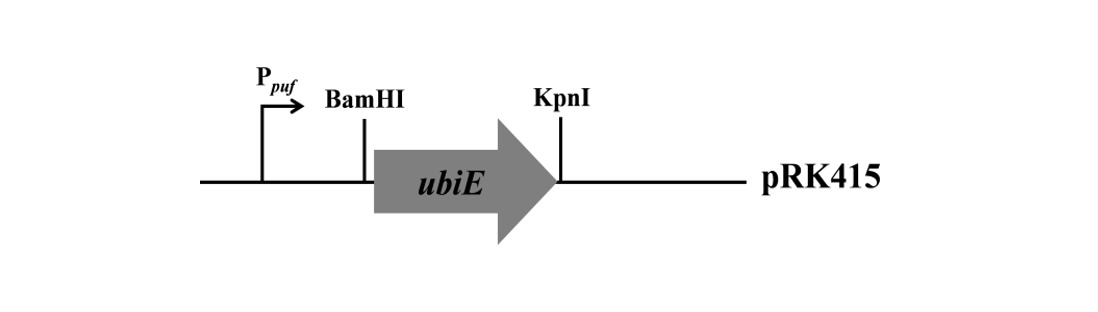
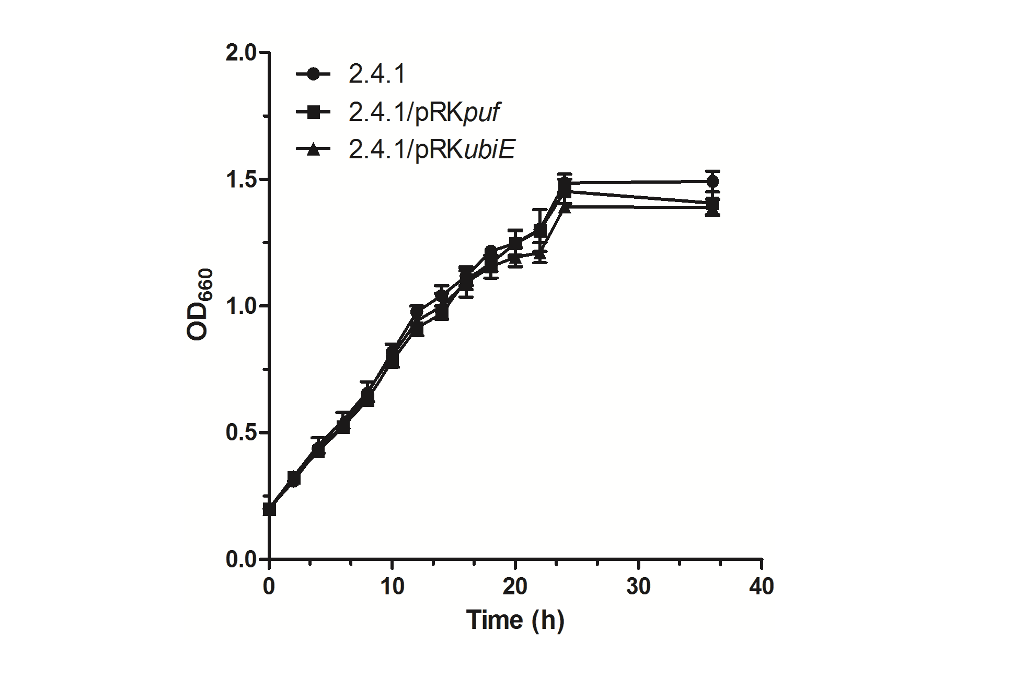
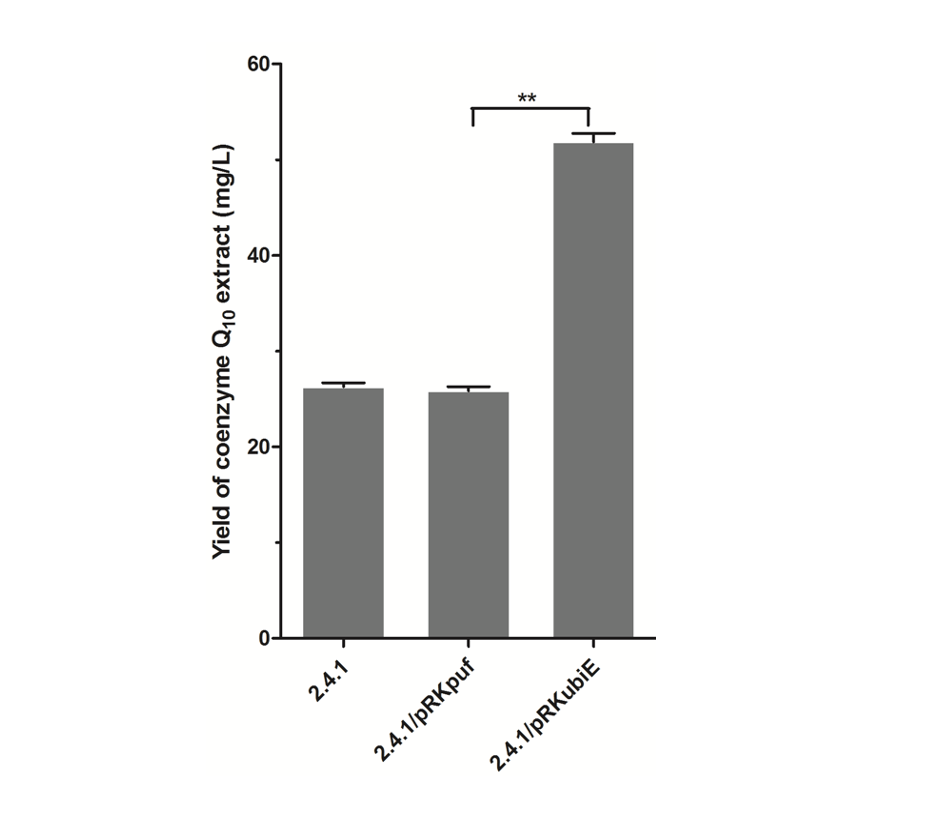
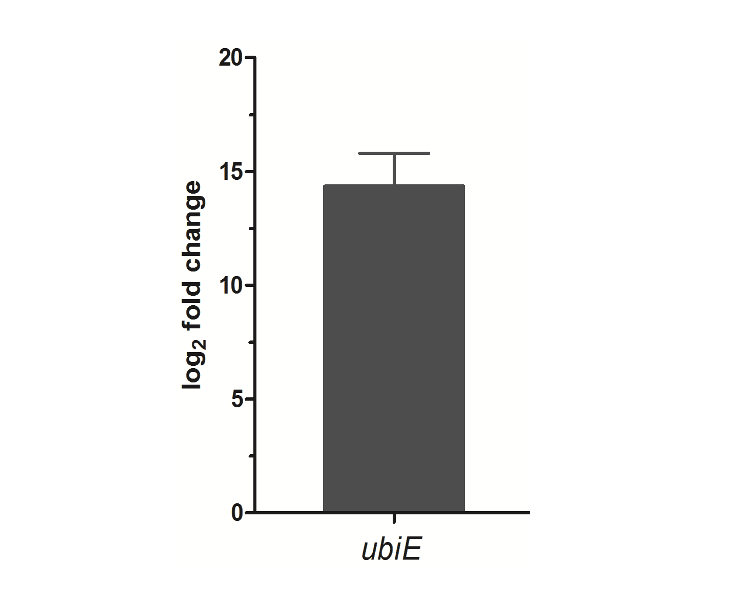
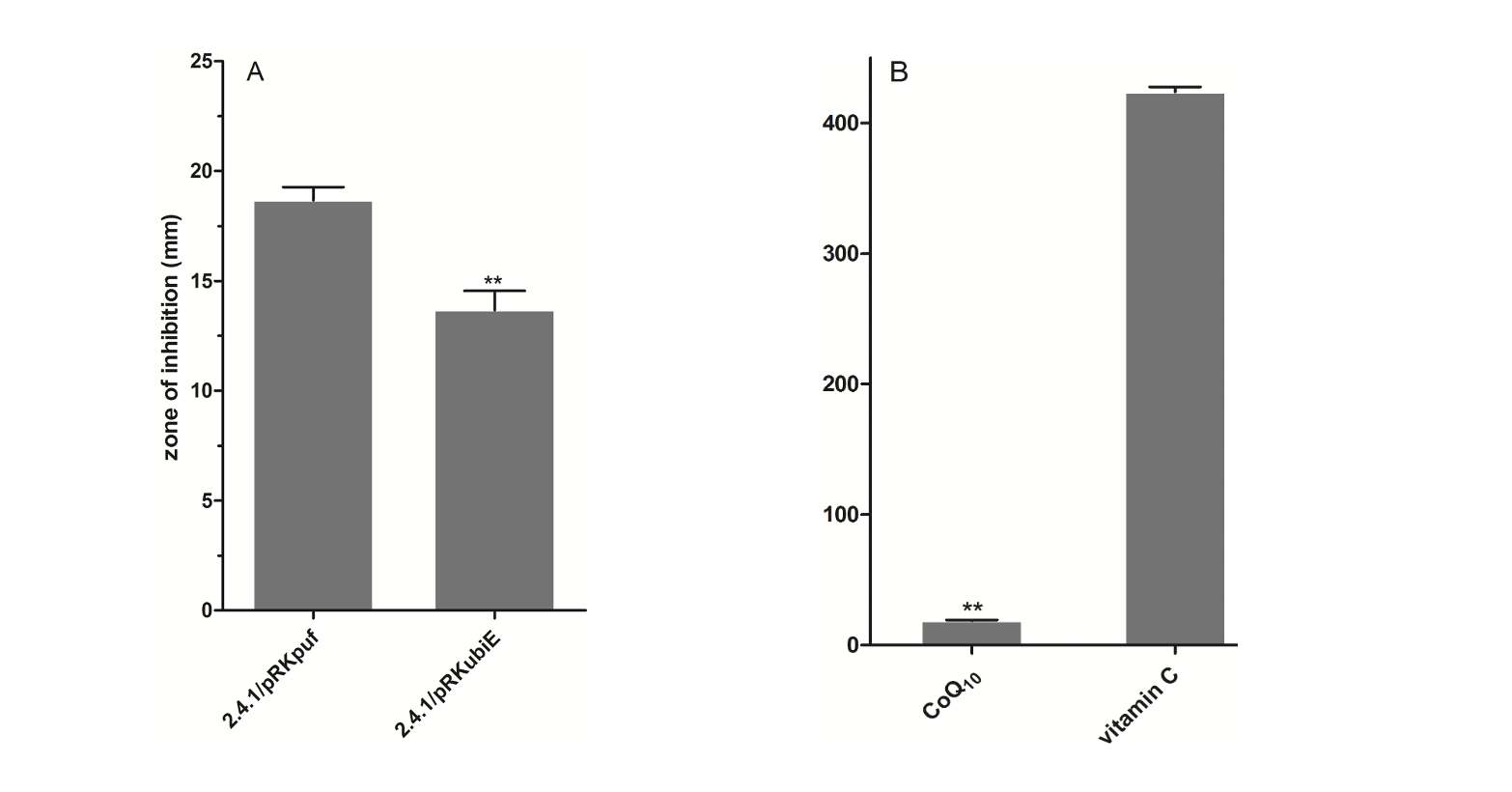
Conclusion
In the present study, we constructed a genetic engineered Rb. sphaeroides for overexpression of ubiE to enhance functional CoQ10 production. The ubiE gene was expressed under the strong puf operon promoter in Rb. sphaeroidesat micro-aerobic growth conditions. Production of the crude CoQ10 from 2.4.1/pRKubiE was enhanced by 80.61%, which was increased much higher than reported literature. The crude CoQ10 exhibited strong hydroxyl radical scavenging activity.
Acknowledgement
This study was supported by Research Project of Sichuan University of Science & Engineering (2015RC27), Open Fund of Meat Processing Key Laboratory of Sichuan Province (16R-27), Sichuan Province Undergraduate Training Programs for Innovation and Entrepreneurship (201610622008). We thank Prof. Dr. Gabriele Klug (Justus-Liebig-University Giessen, Germany) for supplying the expression vector pRKpuf.
Conflict of Interest
No potential conflicts of interest were disclosed.
Author Details
1School of Chemical Engineering, Sichuan University of Science and Engineering, Zigong 643000,
2Meat Processing Key Laboratory of Sichuan Province, Chengdu University, Chengdu, 610106, China
References
Rights and permissions
Open Access: This article is licensed under a Creative Commons Attribution 4.0 International License, which permits use, sharing, adaptation, distribution and reproduction in any medium or format, as long as you give appropriate credit to the original author(s) and the source, provide a link to the Creative Commons license, and indicate if changes were made. The images or other third-party material in this article are included in the article’s Creative Commons license, unless indicated otherwise in a credit line to the material. If material is not included in the article’s Creative Commons license and your intended use is not permitted by statutory regulation or exceeds the permitted use, you will need to obtain permission directly from the copyright holder. To view a copy of this license, visit http://creativecommons.org/licenses/by/4.0/
Cite this Article
DOI: http://doi.org/10.19026/ajfst.17.6012
Sections