Advance Journal of Food Science and Technology
Nutritional Value of Legumes in Relation to Human Health: A Review
Advance Journal of Food Science and Technology 2019 17: 72-85
Cite This ArticleAbstract
The aim of this review was to provide information on the nutritional components of legumes that can improve human wellbeing. Legumes are nutrient dense food crops with numerous health benefits. They have the ability to withstand harsh weather and naturally enrich soil, thus, reducing fertilizing cost. However, despite the advantages of legumes over other crops, they have not been fully utilized in food systems especially in developing countries. This review explores the nutritional profile of different types of legumes, factors influencing legume utilization and, the health benefits of legumes. Thus, providing vital information required to enhance industrial and domestic usage of legumes with a goal to promote legume cultivation for sustainable food production and improved healthy living.
Keywords:
Introduction
Legumes belong to the family Leguminosae and produce seeds within pods (Nieuwenhuis and Nieuwelink, 2005). They are the second most important group of plant crops (after cereals) in human nutrition (Levetin-McMahon, 2008). This is because they are inexpensive to produce and purchase, and have high nutritional properties and beneficial physiological effects (Moreno-Jiménez et al., 2015). Examples of edible legumes include: peas (Pisum sativum), chickpeas (Cicer arietinum L.), lentils (Lens culinaris), soybeans (Glycine max), cowpeas (Vigna unguiculata), bambara groundnuts (Vigna subterranea), pigeon peas (Cajanus cajan), jack beans (Canavalia ensiformis), lupins (Lupinus albus), mung beans (Vigna radiata), yellow peas (Lathyrus aphaca) and black-eyed peas (Vigna unguiculata subsp. unguiculata), peanuts (Arachis hypogaea), dry beans (Phaseolus vulgaris L.), broad beans (Vicia faba), green beans (Phaseolus vulgaris L.), kidney beans (Phaseolus vulgaris) and black turtle beans (Phaseolus vulgaris) (Kouris-Blazos and Belski, 2016).
Legumes are drought-tolerant, adaptable to marginal soils and climates for cultivation and have the ability to absorb the essential nutrient nitrogen from the air and fix it to the soil (Nieuwenhuis and Nieuwelink, 2005; Muzquiz et al., 2012; Mubaiwa et al., 2017). The nitrogen fixing capacity of legumes makes them important crop in tropical areas, where most soils do not contain sufficient nitrogen. This makes legume cultivation an inexpensive and simple way for farmers to enrich the soil (Nieuwenhuis and Nieuwelink, 2005) and reduce their costs for artificial fertilizer. Legumes have the potential to alleviate poverty among small holder farmers during drought and increased weather variability that has been recently experienced in Africa and other developing countries (Mubaiwa et al., 2017). Legumes can also play a vital role in providing nutrition and income for rural low income people in developing countries.
Legumes are rich sources of proteins, carbohydrates and fibres (soluble and insoluble dietary fibres). They are also rich sources of minerals and vitamins such as thiamine, riboflavin and niacin (Kouris-Blazos and Belski, 2016; Stanley and Aguilera, 1985). Legume seed oils can also contribute to essential fatty acids (linoleic and linolenic acids) in human diet. In developing countries, legumes are used to supplement cereals or starchy foods in order to reduce or eliminate protein-energy malnutrition problem in the countries. This is because animal proteins are expensive in these countries.
Many studies have reported that the incorporation of legumes in beverages (Udeozor, 2012; Bolarinwa et al., 2017), snacks (Eneche, 1999; Bolarinwa et al., 2016a) or complimentary foods (Bolarinwa et al., 2016b; Tufa et al., 2016; Abeshu et al., 2016) significantly increased nutrients such as protein, fat and mineral contents in the products. Despite the nutritional importance of legumes, they have received limited usage (both industrially and domestically) in developing countries. Although the average per capita consumption of legumes in developing countries is between 2.3 to 9.9 kg/year (Nedumaran et al., 2015), most legumes (per capita consumption) are used for livestock feed or grown as cover crop to fix nitrogen into the soil. In most cases, legumes are consumed as boiled whole seeds or used to supplement cereal or root and tuber based diets. Reduced utilization of legumes protein could be due to the difficulty in legume processing (Gupta, 1987) and partly due to indigestible carbohydrates in legumes, which causes flatus (responsible for consumers’ discomfort). This review provides information on legume production and consumption, processing and utilization, factors limiting legumes consumptions and utilization, nutrient profile of different legumes, effect of processing methods on the nutritional profile of legumes and health benefits of legumes as quality factors that can enhance industrial utilization and domestic usage of legumes, and increase legume consumption for better health.
Literature Review
Legume production and consumption: Legumes can be classified as pulses (dry edible seeds) or oil seeds (mostly cultivated for their oil contents). Wide varieties of legumes are cultivated in large quantities in many parts of the world. Figure 1 shows the U.S. legume production and export rate from year 2011/12 to 2016/2017. United States legume production increased significantly in terms of production and domestic consumption in 2016/17. Legume export increased from 1.78 billion pounds to 2.79 billion pounds. Even though there was significant increased in U.S. legume production volume over the past several years, there was reduction in the percentage export rate from 51% in 2015/16 to 43% in 2016/17, due to growing domestic consumption (Fig. 1) (Wells and Bond, 2016). Legumes production in 2016/17 in some other countries is also presented in Table 1 (Muthukumarasamy, 2017).
Legume consumption varies from country to country due to factors such as availability of different varieties, environmental and climatic differences, regional and cultural changes associated and domestic time available to cook (Moreno-Jiménez et al., 2015). These factors have led to the development of convenience legume foods such as canned whole legume seeds by industries.
In Latin America, major legumes consumed are common beans of varieties; bayo, pinto, black and peruvian beans. These legumes are mostly processed as canned whole seeds and are the most (82%) preferred by consumers (Morrow, 1991; Rodríguez-Licea et al., 2010). In the Mediterranean, cooked dry legumes of the following varieties; broad beans, chickpeas, split peas, lentils, pinto beans, black-eyed beans, white beans and white lupins are commonly consumed. The daily consumption of legumes in this area is between 8 and 23 g/capita (Kalogeropoulos et al., 2010). In Canada, common beans, chickpeas, lentils and dry peas (Pisum sativum) are mostly cultivated and consumed (Tosh and Yada, 2010).
In Asian countries, mung beans and peas are the most commonly consumed legumes, whereas in African countries, groundnut, cowpea and common bean are the most important legume grains consumed, mostly in processed form rather than as whole boiled legume seeds (Prinyawiwatkul et al., 1996; Morrow, 1991).
Processing and utilization: Legumes are often dried to extend their shelf life and can be cooked and consumed as fresh beans or after drying. In some parts of the world, fresh soybeans in the pod, winged beans, peas, mung beans, lupin, can be cooked and eaten as a side dish or with salads. Soybeans are also roasted whole and consumed or used to prepare soybean butter (Annor et al., 2014). Dried legumes can also be ground into flour and used in food preparation (Iqbal et al., 2006). Legumes are processed into a variety of food products in various countries: tofu (Japan, China), bean sprouts (China, Korea), chilli and refried kidney beans (Mexico), dhal and papadums (India), falafel and hummus (Middle East), navy bean soups (Mediterranean), tempeh (Indonesia), pea soups (Sweden), baked beans and peanut butter (US, Australia) (Kouris-Blazos and Belski, 2016). Whole legume seeds are used to make soups, sauces, beverage (e.g., soymilk), fried and baked products in India, Africa and South America (Annor et al., 2014).
Sprouted legumes are produced from dried legume seeds by allowing seeds to germinate after soaking (Mubarak, 2005). This process helps to increase protein digestibility and mineral bioavailability and also reduce the concentration of anti-nutritional factors (such as tannins, phytic acid) and indigestible carbohydrates (Boye and Ma, 2012). Canned legumes are produced by soaking whole dried legumes followed by blanching and cooking and then packaging in cans with a variety of sauces in order to ease their use in food preparation. Canned legumes especially baked beans are commonly found in supermarkets in the developed countries (Morrow, 1991). Frozen precooked legumes are receiving more attention in the food market for both household use and in the food service sector, due to its convenience (Annor et al., 2014). The potentials of legumes as added flour or as fillings in frozen dough and in processed foods such as bread, pasta, snack foods, soups, cereal bar filing, tortillas and meat, have been investigated (Asif et al., 2013). The results of the investigation showed that the nutritional profile of legume seeds would make them ideal crop for inclusion in snack foods, baby foods and sports foods (Asif et al., 2013).
Research is further exploring novel uses and promising application areas of legumes for industrial application. For instance, several researchers have reported various ways of improving the functionality of legume starch (Joshi et al., 2013; Joshi et al., 2014; Ahmed et al., 2016; Oyeyinka et al., 2017a, 2017b; Leite et al., 2017) and protein (Sun and Arntfield, 2011; Ribotta et al., 2012). However, there is limited information on the use of these starches or proteins for new product development and industrial utilization. Thus, application of modified legume starches and proteins in food systems needs further investigation.
Factors limiting legume consumption and utilization: Two major factors influencing the consumption or utilization of legumes are the anti-nutritional factors and the hard to cook phenomenon. There have been many reviews on the anti-nutritional factors of legumes (Gupta, 1987; Van der Poel, 1990; Jain et al., 2009; Soetan and Oyewole, 2009; Ramadoss and Shunmugam, 2014), but few reports on hard-to-cook feature of legumes.
Hard-to-cook phenomenon in legumes: Despite the nutritional importance of legumes, they received less attention and usage in developing countries due to extensive preparation and cooking requirements. This is so because the middle lamella of legume tissue is composed of pectic substances that are associated with divalent cations such as calcium and magnesium, as well as proteinaceous materials. During cooking, legume cells separate, leading to the removal of the divalent cations from pectinaceous matrix of the middle lamella. This results in enzymatic degradation of phytate by phytase, facilitating cross linking of pectic substances in the middle lamella, thereby causing the formation of calcium pectate and magnesium pectate, which do not solubilize easily during thermal processing, hence causes slower cooking of legumes (Rockland and Jones, 1974).
Another factor that contributes to hard-to-cook-feature of legumes is the deposition of lignin on the middle lamella and cell wall of legume. This normally occurs during storage of legume seeds under conditions of high temperature (>25°C) and high relative humidity (65-75%) for long period (>2 months) (Reyes-Moreno and Paredes-López, 1993; El‐Tabey Shehata, 1992). The presence of phenolic substrates in legume tissues (Duenas et al., 2005; Pirhayati et al., 2011) facilitates the oxidation and polymerization of polyphenolic compounds through cell wall bound peroxidase, resulting in lignin formation (Rockland and Jones, 1974). This is evidence in the findings of Pirhayati et al. (2011), where the hardness of legume seeds stored at 40°C and 73% relative humidity for 6 months increased 3-6 times with corresponding decreased in phytic acids from 36% to 61% and total phenolic from 43% to 61%. The implications of lignification are that it reduces water permeation across cell walls and provides more elasticity to the wall, consequently leading to increased structural integrity. These prevent cell separation during cooking, restrict hydration of legume tissue components (due to hydrophobic nature of lignin) and reduce starch gelatinization, thereby causing hardened texture (Rockland and Jones, 1974).
The degree of legume hardness however depends on factors such as legume cotyledon, seed coats or shell (El‐Tabey Shehata, 1992; Reyes-Moreno et al., 2000). Hardness of legume seed shells has been classified into two (2) groups, namely; reversible (commonly found in freshly harvested legume) and irreversible (develops during long storage period). Development of hard shell in freshly harvested legumes has been attributed to factors such as genetics, climatic conditions, crop husbandry, seed size and degree of ripeness (El‐Tabey Shehata, 1992).
The hard-to-cook characteristic of legume makes the seeds not to soften adequately during soaking and not to become tender after prolong cooking time (Pirhayati et al., 2011). Apart from reducing the cooking ability of legume, hard-to-cook property reduces the availability of legume amino acid after cooking and also reduces its acceptance by consumer (Reyes-Moreno and Paredes-López, 1993).
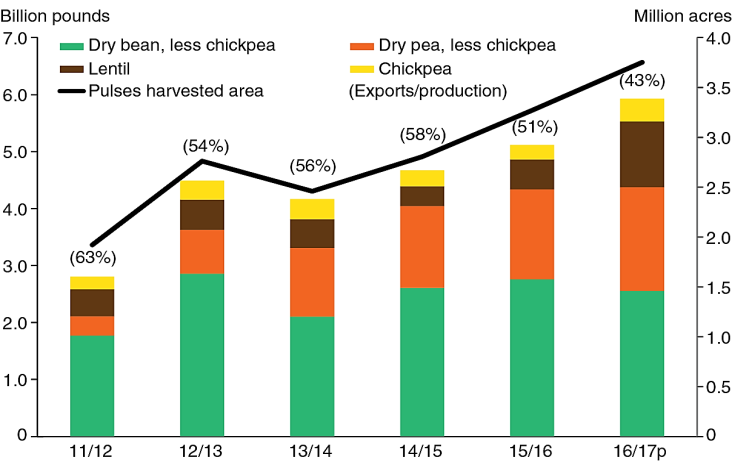
Legume types by country | Production year and quantity in million MT 2016/17 |
---|---|
Canada | |
Lentils | 3.20 |
Peas | 4.80 |
Australia | |
Chickpeas | 1.12 |
Lentils | 0.50 |
India chickpeas, pigeon peas, black gram, green gram, lentils, peas, beans, moth beans and horse gram | 20.8 |
Myanmar pigeon pea, black gram, green gram | 6.5 |
Africa (mainly Tanzania, Mozambique, Malawi, Kenya) Pigeon peas | 4.3 |
Muthukumarasamy (2017) |
Profile of Legumes
The three major macronutrients profiles of legumes are presented in Table 2.
Protein: Legumes are important sources of inexpensive protein for human and animal nutrition. It is the richest sources of plant protein, providing about 10% of the total dietary protein requirements for human world-wide (Asif et al., 2013). Protein content of legume (17-37%) is similar to that of red meat (22-31%) (Chan et al., 1995) but higher than that of milk (3.26-5.73%) (Barłowska et al., 2011), cereals (5.8-15%) (Shewry, 2007) and root and tubers (2.28-4.04%) (Ceballos et al., 2006). This indicates that legume based diets can supply human body with the recommended protein intake at less cost compared to animal proteins. The protein quality of legumes as revealed by various studies showed that all legume proteins apart from soybeans (Table 3) are incomplete because they contain relatively low amounts of sulphur containing amino acids (methinine, cysteine) which are higher in cereals. On the other hand, cereals are lower in lysine (one of the essential amino acid). The levels of essential amino acids in legume seeds compared well with the FAO/WHO/UNU amino acid scoring pattern for adults and children (FAO/WHO/UNU, 1985). This indicates that regular consumption of legumes especially as boiled whole seeds or as protein supplementation in cereal diets can reduce protein malnutrition, a major problem in developing countries (Iqbal et al., 2006).
Carbohydrate: The total carbohydrate content of legumes ranges from 11-63% (Table 2), depending on the type of legume and cultivar, with its major components being starch (35-55%), in addition to monosaccharide, oligosaccharide and non-starch polysaccharide (Stanley and Aguilera, 1985; El-Adawy, 2002; Mubarak, 2005). Legumes are relatively high in dietary fibre (cellulose, hemicellulose, lignin and β-glucans) (Stanley and Aguilera, 1985). Dietary fibre plays important role in food digestibility and bioavailability of nutrients. Soluble dietary fibre and insoluble dietary fibre values of legumes ranges from 0.0-4.6% and 13-30%, respectively (Table 2). Both soluble and insoluble dietary fibres have nutritional benefits. Soluble fibre promotes growth of good bacteria (because probiotics prefer shorter chain soluble fibre), help to regulate glucose level in the blood and also reduces blood cholesterol, while insoluble fibre promotes movement of food materials through the digestive system and also support the growth of probiotics bacterial (intestinal microflora) (Tosh and Yada, 2010; De Almeida Costa et al., 2006; Lin and Lai, 2006). As presented in Table 2, chickpea has the highest total carbohydrate but contains the lowest amount of soluble and insoluble fibre (De Almeida Costa et al., 2006). Fibre and carbohydrate contents of other legumes are also shown in Table 2. In addition to fibre, legumes are also good sources of resistance starch, which has a role in human nutrition similar to that of dietary fibre.
Lipid: The lipid profiles of legume seeds show that they are generally low in fat (Table 2). The major fatty acids in legumes are: linoleic, oleic, palmitic and linolenic acid. Among the legume types, chickpea has the highest linoleic acid (polyunsaturated fat) followed by soybean and lentil. However, soybean has the highest oleic acid (monounsaturated fat) compared to chickpea, lentil and all other legumes (Table 4). Cowpea and mung bean are superior to soybean, chickpea and other legumes in terms of omega-3 fatty acid (linolenic acid), while soybean has the highest content of omega-6 fatty acid (linoleic acid). Although omega-3 fatty acid has been reported to inhibit platelet aggregation and inflammatory cytokine production, with the potential of reducing risk of cardiovascular disease (Nozue et al., 2013), the levels of this fatty acid in legumes may be too low to exhibit this effect. However, legumes can be used to complement other foods that are relatively high in omega-3 fatty acids.
Minerals: The mineral content of legumes varies, depending on the legume type and cultivar. Winged bean has the highest calcium content (80-370 mg/100 g) followed by chickpea (57-197 mg/100 g) and soybean (180 mg/100 g), whereas lentil (73-120 mg/100 g) and lupin (84 mg/100 g) have the lowest calcium values (Table 2). All the legumes contain high amounts of phosphorus. Potassium (1800 mg/100 g) and magnesium (280 mg/100 g) are highest in soybeans, whereas winged bean has the highest iron content (2-18 mg/100 g) while zinc is highest (1-6.8 mg/100 g) in chickpea. Since cereals and tuber-based diets are low in iron and zinc (FAO/WHO, 2002), addition of legumes to cereals or tuber-based foods will improve the mineral content of this food combination.
Vitamins: Vitamins are important micromolecules that are required in small but adequate quantities to maintain optimal human health. As presented in Table 2, lupin has higher amount of vitamin B1 (thiamin) and B2 (riboflavin), soybean has the highest content of vitamin B3 (niacin) and folate, while vitamin B6 (pyridoxine) is higher in lentil, whereas, chickpea is rich in vitamin C (ascorbic acid). Thiamin levels in most legumes are within the Recommended Dietary Allowance (RDA) for children and adults (FAO/WHO, 2002). Riboflavin levels in mung beans, winged beans and soybeans are within the RDA for children while riboflavin level in lupin is same as the RDA for adolescent (Table 2). The levels of pyridoxine in chickpeas and lentils (Table 2) are within the range for children. Although legumes are not regarded as major sources of ascorbic acid (Vitamin C), the level of ascorbic acid in chickpeas and folate levels in chickpeas and lentils are within the RDA for children (Table 2) (IOM, 2000), while folate levels in soybeans are within the range for adults (Table 2) (IOM, 1998). The information provided in this section shows that most legumes can meet up the B-vitamins requirements of children and can complements adults’ vitamins intake.
Antioxidants: Besides the high protein and mineral contents of legumes, they are also rich sources of phenolic compounds. Phenolic compounds are natural antioxidants, representing important group of bioactive compounds in foods. Phenolic compounds are plant secondary metabolites that are mostly present in high concentration in legume seed coats (with lower concentration in the cotyledon). Generally, phenolic compounds have minimum of one aromatic ring with one or more hydroxyl groups attached and can be classified as phenolic acids, flavones, flavanones, isoflavones, flavonols, anthocyanins and tannins (Del Rio et al., 2013). Phenolic acid and flavonoids represent the major components of legume phenolic compounds. Flavonoids have many health-related functions, including anti-neoplastic activity and radio-resistance activity (Shi et al., 2016). On the other hand, tannin and anthocyanin concentration in legumes determine the colour of legume seeds.
Legume phenolic compounds have significant antioxidant capacity with beneficial implication in human, by reducing oxidative stress and cancer. They help in the management of type-II diabetes due to their ability to inhibit enzymes related to glucose absorption (Faris et al., 2009; Mojica et al., 2015). Legume phenolic compounds also provide protection against oxidative damage, they have anti-mutagenic activity and protect human body from disease (Duenas et al., 2005). As shown in Table 2, soybeans have the highest amounts of total phenolic (2850-8500 mg catechin equivalent/g) and flavonoid (320-1900 mg catechin equivalent/g) with antioxidant capacity of 500-7800 mg/L (Lin and Lai, 2006). This indicates that soybeans contain high contents of bioactive compounds with high antioxidative ability. Thus, regular consumption of soybeans will be beneficial to human health. However, mung bean seed has flavonoid contents of 6.03 mg gallic acid/g, with antioxidant capacity of 4120 mg/L (Chandrasiri et al., 2016). Among the legumes reviewed in this study, lupin has the lowest total phenolic content (0.19 mg/g) while lentil has the lowest (2.21 mg/g) flavonoid content. Variation in the phenolic compounds of legumes may influence their free radical scavenging activity.
In addition to phenolic compounds, tocopherol also contributes to the antioxidant activity of legumes. Legumes contain four major tocopherol isomers (α-, β-, γ- and 𝛿-tocopherol) (Table 2). Although the levels of tocopherol in legumes are low, their content in legumes is higher than in cereals (Bouchenak and Lamri-Senhadji, 2013). Legumes tocopherols, especially γ-tocopherol have been reported to be protective against cardiovascular disease (Amarowicz and Pegg, 2008). In addition, tocopherol also contributes to shelf life stability of legume seed oil because of its antioxidant activity. According to Amarowicz and Pegg (2008) and Grela and Gunter (1995), the average value of γ-tocopherol in legume samples varied from 0.43 mg/100 g (cowpea) to 23.8 mg/100 g (soybean). This is an indication that tocopherol content is not equally distributed in all legumes, but depends on legume species and variety.
Parameters | Mung beans (Vignaradiata) | Winged beans (Psophocarpustet ragonolobus) | Cowpea (Vignaunguiculata) | Lupin* (Lupinusalbus) |
---|---|---|---|---|
Energy (kcals) | 306.00-415.001 | 402.00-490.001 | - | 332.002 |
Protein (%) | 22.60-27.501,7 | 29.101 | 21.70-24.7010,11,12 | 15.80-37.002,8 |
Moisture (%) | 9.80-15.201,7 | 11.201 | 8.90-11.2010,11,12 | - |
Carbohydrate (%) | 53.30-62.301,7 | 31.601 | 59.90-62.7011,12 | 11.002 |
Fibre (%) | 3.80-4.701,7 | 6.801 | 2.301 | 33.002 |
Soluble dietary fibre (%) | 0.11-0.8619,21 | - | 0.77-0.8923 | 1.6124 |
Insoluble dietary fibre (%) | 13.07-27.8519,21 | - | 10.20-15.5023 | 30.0324 |
Total dietary fibre (%) | 13.93-14.5719 | - | 11.09-16.1723 | - |
Ash (%) | 3.40-3.801,7 | 3.601 | 2.90-4.2010,11,12 | - |
Fat (%) | 0.40-1.871,7 | 17.701 | 0.89-4.8010,11 | 7.902 |
Saturated (%) | 40.006 | 21.9013 | 30.2014 | 1.50-14.502,8 |
Polyunsaturated (%) | 55.306 | 36.9013 | 60.5014 | 3.80-28.92,8 |
Omega 3 linolenic (%) | 19.2.006 | 1.6013 | - | |
Monounsaturated (%) | 4.906 | 38.6013 | - | 2.60-53.902,8 |
Calcium (mg/100g) | 84.00-135.001,7 | 80.00-370.001,26 | 176.0010 | 84.002 |
Phosphorus (mg/100g) | 360.00-430.001,7 | 200.00-610.001,20 | 303.0010 | 440*1 |
Iron (mg/100g) | 8.00-13.501,7 | 2.00-18.001,26 | 2.6010 | 4.902 |
Magnesium(mg/100g) | 5.10-5.607,15 | 34.0-25526,*1 | 4.8010 | 18.902 |
Potassium(mg/100g) | 1.16-3.627,15 | 1110-180026 | 1.2810 | 810.002 |
Zinc(mg/100g) | 0.25-0.4715 | 3.10-5.0026 | 5.1010 | 3.602 |
Vit B-1 (mg/100g) | 0.12-0.7025 (0.5-1.5)5 | 0.08-1.7026 | - 3.002 | |
Vit B-2 (mg/100g) | 0.23-0.4725 (0.5-1.3)5 | 0.20-0.5026 | - | 1.002 |
Vit B-3 (mg/100g) | 1.10-3.1025 (2.0-16)5 | 3.10-4.6026 | - | 1.902 |
Vit B-6 (mg/100g) | (0.1-1.7)5 | 0.10-0.2526 | - | 0.102 |
Vit C (mg/100g) | 6.27-10.0016,25 (15-90)11 | Trace26 | - | 0.002 |
Folate (µg/100g) | (150-400)l | 25.60-63.5026 | - | - |
Total phenolics (mg gallic acid equivalent/g) | 2.21-6.036,21 | - | 16020 | 0.198 |
Total flavonoids (mg catechin equivalent/g) | 0.06-22.696,21 | - | - | 630.708 |
Tocopherol (mg/100g) | ||||
α-tocopherol | 0.11-1.0115 | - | - | - |
β-tocopherol | - | - | - | 0.45#8 |
γ-tocopherol | 6.07-8.0915 | 22.826 | 0.4316 | - |
𝛿-tocopherol | 0.46-1.1215 | - | 1.8316 | 0.028 |
Parameters | Soybeans (Glycine max) | Chickpeas (Cicer arietinum L.) | Lentil (Lens culinaris) | |
Energy (kcals) | 398.002 | 378.004 | 286.00-432.902,8 | |
Protein (%) | 31.00-34.002,3 | 20.50-24.004,10 | 6.10-26.102,3,8, 10 | |
Moisture (%) | 10.003 | 7.3010 | 9.30-11.103, 10 | |
Carbohydrate (%) | 13.002 | 63.004 | 16.20-35.002,8 | |
Fibre (%) | 20.002 | 12.204 | 7.80-14.001,8 | |
Soluble dietary fibre (%) | 1.53-4.6319 | 0.00-0.0022 | 1.37-1.4422 | |
Insoluble dietary fibre (%) | 19.36-26.3219 | 13.90-15.4022 | 19.00-21.4022 | |
Total dietary fibre (%) | 9.76-15.0719 | - | - | |
Ash (%) | 4.703 | 3.6010 | 0.81-3.703,8,10 | |
Fat (%) | 17.70-20.002,3 | 3.10-6.044,8,10 | 1.50-3.202,3,10 | |
Saturated (%) | 2.902 | 0.30-0.604,5 | 0.05-0.304,5 | |
Polyunsaturated (%) | 12.502 | 0.30-2.732,4 | 1.002 | |
Omega 3 linolenic (%) | 1.402 | 0.002 | 0.002 | |
Monounsaturated (%) | 3.002 |
0.10-1.342,4 | 0.302 | |
Calcium (mg/100g) | 180.002 | 57.00-197.004,10 | 73.00-120.002,10 | |
Phosphorus (mg/100g) | 704*1 | 252.00-360.004,5,10 | 180.00-294.005,10 | |
Iron (mg/100g) | 9.52 | 1.80-4.312,4,10 | 3.1010 | |
Magnesium(mg/100g) | 23.0-2802, *1 | 4.80-7.904,5 | 8.202 | |
Potassium(mg/100g) | 1800.002 | 264.00-1155.004,10 | 840.00-874.002,10 | |
Zinc(mg/100g) | 4.002 | 1.00-6.802,4,5,10 | 1.30-3.002,5 | |
Vit B-1 (mg/100g) | 0.762 | 0.32-0.462,4.9 | 0.402 | |
Vit B-2 (mg/100g) | 0.602 | 0.21-0.402,4,9 | 0.162 | |
Vit B-3 (mg/100g) | 2.702 | 2.302 | 2.302 | |
Vit B-6 (mg/100g) | 0.402 | 0.20-0.552,4 | 0.602 | |
Vit C (mg/100g) | 0.002 | 33.002 | 3.002 | |
Folate (µg/100g) | 375.002 | 56.00-172.002,5 | 111-1812,5 | |
Total phenolics (mg gallic acid equivalent/g) | 2850-850019 | 0.21-1.678,16 | 0.26-4.178,16 | |
Total flavonoids (mg catechin equivalent/g) | 320-190019 | 0.18-3.1616 | 0.72-2.2116 | |
Tocopherol (mg/100g) | ||||
α-tocopherol | 6.5518 | 0.07-6.98,16,17 | 0.38-1.616,17,18 | |
β-tocopherol | - | 1.49-5.5#8,16,17 | 0.19-0.3816 | |
γ-tocopherol | 23.7618 | - | 4.66-6.6516,18 | |
𝛿-tocopherol | 6.2418 | 0.228 | 0.20-0.2716 | |
1[Rehman and Shah, 2005]. 2[Kouris-Blazos and Belski, 2016]. 3[Stanley and Aguilera, 1985]. 4[Annor et al., 2014]. 5[Iqbal et al., 2006]. 6[Alajaji and El-Adawy, 2006]. 7[Nechuta et al., 2012]. 8[Wang et al., 2013]. 9[Wells and Bond, 2016]. 10[Braakhuis et al., 2016]. 11[Boye and Ma, 2012]. 12[Asif et al., 2013]. 13[Joshi et al., 2013]. 14[Joshi et al., 2014]. 15[Ahmed et al., 2016]. 16[Moongngarm and Saetung, 2010]. 17[Oyeyinka et al., 2017a]. 18[Khalil and Mansour, 1995]. 19[Morrow, 1991]. 20[Patil and Khan, 2011]. 21[Moongngarm et al., 2014]. 22[Muthukumarasamy, 2017]. 23[Oyeyinka et al., 2017b]. 24[Leite et al., 2017]. 25[Sun and Arntfield, 2011]. 26[Ribotta et al., 2012]; (-) indicates not available. (*) indicates Lupin flour. (#) indicates β + γ; Values in parenthesis are RDA values of vitamins for children and adults. (*1) USDA, 2018 |
Amino acid | Mung bean | Chickpeas | Cowpea | Lentil |
---|---|---|---|---|
Tyrosine | 3.30-901,2,3 | 2.80-5.203,4 | 3.00-3.204,9 | 7.50-32.003,4 |
Phenylalanine* | 5.70-11.001,2,3 | 5.50-11.003,4 | 6.20-7.504,8,9 | 5.00-8.003,4 |
Threonine* | 3.10-8.901,2,3 | 3.10-7.403,4 | 3.80-4.504,8,9 | 3.50-9.403,4 |
Cystine | 0.751 | 0.604 | 0.50-1.014,9 | 0.90d |
Methionine* | 1.92-3.101,3 | 1.10-3.303,4 | 2.20-2.304,8,9 | 0.80-2.103,4 |
Leucine* | 8.36-19.801,2,3 | 8.70-15.003,4 | 7.70-8.504,8,9 | 7.80-13.503,4 |
Isoleucine* | 4.74-11.901,2,3 | 4.80-8.003,4 | 4.50-4.804,8,9 | 4.10-7.903,4 |
Lysine* | 4.19-19.501,2,3 | 7.20-15.503,4 | 6.60-8.104,8,9 | 7.00-16.303,4 |
Valine* | 5.20-16.901,2,3 | 4.60-7.803,4 | 5.00-5.704,8,9 | 5.00-9.703,4 |
Tryptophan* | 0.97-2.201,3 | 0.90-2.503,4 | 0.70-3.604,8,9 | 0.70-2.503,4 |
Aspartic acid | 13.50-27.601,2,3 | 11.00-27.703,4 | 10.80-12.904,8,9 | 11.80-24.503,4 |
Glutamic acid | 21.70-42.101,2,3 | 17.30-40.103,4 | 17.20-18.704,8,9 | 21.50-38.603,4 |
Proline | 4.23-9.201,2,3 | 3.80-8.903,4 | 4.00-4.904,8,9 | 3.50-8.503,4 |
Serine | 4.95-15.301,2,3 | 3.70-11.103,4 | 4.50-5.604,8,9 | 5.20-11.203,4 |
Glycine | 4.26-8.501,2,3 | 3.70-8.203,4 | 3.80-4.604,8,9 | 3.60-9.003,4 |
Alanine | 4.35-12.901,2,3 | 4.97-8.503,4 | 4.20-4.804,8,9 | 4.20-10.603,4 |
Arginine* | 6.33-19.601,2,3 | 8.30-17.003,4 | 7.50-8.004,8,9 | 7.80-15.003,4 |
Histidine* | 2.49-9.501,2,3 | 3.00-5.303,4 | 3.10-4.704,8,9 | 2.20-4.803,4 |
Amino acid | Soybean | Winged bean | Faba bean | |
Tyrosine | 3.20-4.005,12 | 3.20-4.406,12 | 3.60-7.503,7 | |
Phenylalanine* | 4.80-5.305,10,12 | 3.90-5.806,11,12 | 4.20-7.103,7 | |
Threonine* | 3.20-4.005,10,12 | 3.40-4.306,11,12 | 4.10-9.003,7 | |
Cystine | 0.80-1.505,10,12 | 1.40-1.606,12 | 1.307 | |
Methionine* | 0.67-2.505,10,12 | 0.70-1.206,11,12 | 1.10-1.803,7 | |
Leucine* | 7.30-7.805,10,12 | 7.50-9.006,11,12 | 7.20-14.203,7 | |
Isoleucine* | 3.80-5.805,10,12 | 3.90-4.906,11,12 | 3.30-7.803,7 | |
Lysine* | 6.60-7.005,10,12 | 6.10-8.006,11,12 | 7.30-15.803,7 | |
Valine* | 4.40-5.205,10,12 | 4.60-4.906,11,12 | 3.70-9.503,7 | |
Tryptophan* | 1.20-1.9010,12 | 0.6011 | 1.10-2.603,7 | |
Aspartic acid | 11.70-12.205,12 | 10.50-11.506,12 | 12.90-24.203,7 | |
Glutamic acid | 17.70-18.505,12 | 13.30-15.306,12 | 15.80-40.503,7 | |
Proline | 5.10-5.405,12 | 6.10-6.906,12 | 4.90-10.003,7 | |
Serine | 4.60-5.605,12 | 4.90-5.606,12 | 5.80-11.303,7 | |
Glycine | 3.80-4.505,12 | 4.10-4.306,12 | 4.70-10.003,7 | |
Alanine | 4.30-4.505,12 | 4.20-4.306,12 | 5.10-10.803,7 | |
Arginine* | 7.00-8.805,12 | 5.10-6.506,11,12 | 10.70-16.303,7 | |
Histidine* | 2.50-2.705,12 | 2.30-2.706,11,12 | 3.20-6.603,7 | |
1[Messina, 2014]. 2[Alajaji and El-Adawy, 2006]. 3[Chan et al., 1995]. 4[Braakhuis et al., 2016]. 5[Barłowska et al., 2011]. 6[Shewry, 2007]. 7[Ceballos et al., 2006]. 8[FAO/WHO/UNU, 1985]. 9[De Almeida Costa et al., 2006]. 10[Lin and Lai, 2006]. 11[Nozue et al., 2013]; 12[FAO/WHO, 2002]; (*) indicates essential amino acid |
Fatty acid (% of total fatty acid) | Mung bean | Chickpeas | Cowpea | Lentil |
---|---|---|---|---|
12:0 Lauric | - | - | 0.0614 | - |
14:0 Myristic acid | 0.0115 | 0.17-0.314,13 | 0.2014 | 0.14-0.408,10 |
16:0 Palmitic acid | 27.80-32.402,15 | 10.51-20.404,7,11,12 | 25.10-35.929,14 | 14.34-17.907,8,10,12 |
16:1 Palmitoleic acid | - | 0.18-0.504,7,11 | 0.3614 | 0.09-0.627,10 |
18:0 Stearic acid | 1.70-7.502,15 | 1.40-9.204,8,11,12 | 5.48-7.269,14 | 1.24-3.007,8,10,12 |
18:1 Oleic acid | 2.10-4.902,15 | 22.20-34.808,11,12 | 7.98-10.479,14 | 20.11-37.607,8,10,12 |
18:2 Linoleic acid | 34.50-36.102,15 | 46.80-60.084,8,11,12 | 20.64-31.709,14 | 43.30-47.177,10,12 |
18:3 Linolenic acid | 19.20-21.402,15 | 0.90-2.744, 8, 11,12 | 18.80-23.499,14 | 6.90-13.287,8,10,12 |
20:0 Eicosanoicacid | 0.2315 | 0.60-1.004,8, 11,13 | 2.0914 | 0.44-0.867,10 |
22:0 Behenic acid | 0.1515 | 0.274 | 5.6614 | 0.28-0.517,10 |
22:1Erucic | - | 0.32-0.3413 | 0.1414 | 0.1410 |
Fatty acid (% of total fatty acid) | Soybean | Winged bean | Pigeon pea | |
12:0 Lauric | 0.106,14 | 0.116 | 0.106 | |
14:0 Myristic acid | 0.20-0.906,7 | 0.061,16 |
0.206 | |
16:0 Palmitic acid | 10.00-18.201,3,5,6,12 | 9.72-12.701,12 | 10.98-21.406,17 | |
16:1 Palmitoleic acid | 0.20-0.686,14 | 0.831 | 0.306 | |
18:0 Stearic acid | 2.30-5.601,3,5,6,12 | 4.10-5.691,16 | 3.32-7.606,17 | |
18:1 Oleic acid | 22.60-52.001,2,5,6,12 | 29.80-39.001,16 | 1.60-8.446,17 | |
18:2 Linoleic acid | 47.20-59.501,3,5,6,12 | 27.17-32.201,16 | 22.54-54.806,17 | |
18:3 Linolenic acid | 5.10-11.001,3,5,6,12 | 1.80-2.021,16 | 5.606 | |
20:0 Eicosanoicacid | 0.20-2.091,6,14 | 1.00-2.021,16 | 0.26 | |
22:0 Behenic acid | 0.301 | 8.30-13.381,16 | - | |
22:1Erucic | Tr6 | 0.5016 | 2.506 | |
1[FAO/WHO, 2002]. 2[Alajaji and El-Adawy, 2006]. 3[IOM, 2000]. 4[IOM, 1998]. 5[Del Rio et al., 2013]. 6[Shi et al., 2016]. 7[Oyeyinka et al., 2017a]. 8[Khalil and Mansour, 1995]. 9[Faris et al., 2009]. 10[Mojica et al., 2015]. 11[Duenas et al., 2005]. 12[Chandrasiri et al., 2016]. 13[Bouchenak and Lamri-Senhadji, 2013]. 14[Joshi et al., 2014]. 15[Ahmed et al., 2016]. 16[Joshi et al., 2013]. 17[Amarowicz and Pegg, 2008]; (-) indicates not available; (Tr) indicates trace amount (<0.05%) |
Effects of Processing Methods on the Nutritional Composition of Legumes
Processing methods have been used for decades to reduce anti-nutritional factors and increase the nutritive value of legume seeds. Cooking methods such as boiling, autoclaving and microwave cooking, and germination have been reported to have no significant effect on the total protein content of chickpea, but increased its crude fibre by 21-30% (El-Adawy, 2002; Alajaji and El-Adawy, 2006) and significantly decreased non-protein nitrogen, carbohydrate fractions (reducing sugars, sucrose, raffinose and stachyose), fat, ash, minerals and B-vitamins contents of chickpeas and mung beans (El-Adawy, 2002; Alajaji and El-Adawy, 2006; Mubarak, 2005). All processing methods (dehulling, soaking, boiling, autoclaving and microwave cooking and germination) decreased concentrations of lysine, tryptophan, threonine and sulfur-containing amino acids in legumes (El-Adawy, 2002; Alajaji and El-Adawy, 2006; Mubarak, 2005; Hefnawy, 2011).
Germination is a process by which wet grain or seeds are allowed to sprout to 0.5-1.0 mm long (Patil and Khan, 2011). During the process of germination, a number of biochemical processes takes place due to the activation of various enzymes leading to change in nutritional quality and chemical compositions of the germinated seed (Moongngarm et al., 2014). Germination can increase bioactive compounds including antioxidants such as ascorbic acid, tocopherols and tocotrienols in grains or seeds (Moongngarm and Saetung, 2010). Germination was reported to be better for minerals and B-vitamins retention in legumes compared to cooking methods (microwave cooking, boiling and autoclaving) possibly because of its ability to degrade phytic acids in seeds and thus increased the availability of minerals in germinated seeds (El-Adawy, 2002). Germination increased vitamin B2 contents of chickpea seeds by 16% compared to cooking methods, while boiling, autoclaving and microwave cooking reduced it by 51, 48 and 42%, respectively (El-Adawy, 2002). Similarly, germinated faba beans were reported to contain 21% riboflavin more than the non-germinated faba beans. In another study, vitamin B9 levels in germinated soybeans and mung beans were reported to increase by 77 to 274% and 91 to 234%, respectively (Gan et al., 2017). Among the cooking methods, retention of vitamin B1 in legume cooked by microwaving was higher (42.4%) than those by boiling (33.8%) and autoclaving (35.5%) (El-Adawy, 2002). On the other hand, mineral retention was higher (97-109%) in germinated mung bean seeds than in boiled (68-94%), autoclaved (76-95%) and microwave cooked (68-93%) mung bean seeds. However, all processing methods improved In vitro protein digestibility and protein efficiency ratio in legume by 4-6% and 6-9%, respectively (El-Adawy, 2002; Mubarak, 2005).
Cooking methods were more effective in reducing legumes anti-nutritional factors such as trypsin inhibitor (81-95%), haemagglutinin activity (100%), chymotrypsin inhibitors (100%), saponins (44-53%), tannins (30-57%) and phytic acid (29-55%) (El-Adawy, 2002; Mubarak, 2005; Alajaji and El-Adawy, 2006; Hefnawy, 2011; Sathya and Siddhuraju, 2015) than dehulling (8-33%) and soaking (16-49%) (Mubarak, 2005). On the other hand, germination and fermentation are more effective in reducing phytate (75-95%) (Mubarak, 2005), while fermentation process is effective in reducing trypsin inhibitor (99%), protease inhibitor (98%) and tannins (94-257%) in legumes (Adeyemo and Onilude, 2013; Sathya and Siddhuraju, 2015). For instance, germination was reported to reduce phytate contents of mung beans from 15.8 to 4.0% (Mubarak, 2005) while fermentation was reported to reduce phytate content of soybeans from 1.16 to 0.04 mg/g (Adeyemo and Onilude, 2013). Significant reduction in phytate levels of germinated or fermented legume seeds could be due to activation of phytase during germination or fermentation process. In another study, dry heating of legume increased its protein and lipid contents by 7% and 11%, respectively, while fermentation resulted in 35% and 43% increment in legume protein and lipid contents, respectively (Sathya and Siddhuraju, 2015). Germinated soybeans was reported to show increased in total crude protein (21%), ascorbic acid (21-25%) (Bau et al., 1997), riboflavin (52%), niacin content (18%) and aspartic acid level (52%) (Gan et al., 2017), but decreased in lysine (55%) and lipase inhibitor activity (18%) compared to non-germinated soybeans (Bau et al., 1997).
Apart from direct cooking, combined effects of pre-treatments and cooking methods on the nutritional composition of legumes have also been studied. Autoclave cooking and boiling of legumes after soaking for 4 h at room temperature was reported to significantly increase protein and starch digestibility of legumes, with autoclaved legumes having the maximum improvement in protein (95.7-105%) and starch (117-138%) digestibility compared to 86.0-93.3% and 84.0-90.4% for boiled legumes protein and starch digestibility, respectively (Rehman and Shah, 2005). Similarly, soaking legumes in ash, sodium bicarbonate, sugar or water prior to autoclaving increased the protein and lipid contents of the legumes by 7-23% and 62-67%, respectively, but decreased other proximate components (ash, carbohydrate, crude fibre) of the legumes (Sathya and Siddhuraju, 2015).
In general, cooking methods such as soaking, boiling, autoclaving and microwave cooking, do not have significant effect on legume proteins but improve their starch and protein digestibility and in some cases increased the crude fibre and lipid contents. However, germination and fermentation are the best method in terms of protein increment and for minerals and vitamins retention. Thus, germination or fermentation of legumes prior to flour preparation would improve its nutrient content. Among the cooking methods, microwave is the best in terms of nutrient retention. Thus, microwave cooking may be preferred for preparation of whole seed legumes. All processing methods are suitable for reduction of anti-nutritional factors in legumes.
Legumes and health: Several epidemiological studies have reported that regular consumption of legumes reduce susceptibility of individuals to chronic disease such as cardiovascular disease (through prevention of its associated risk factor; cholesterol, blood pressure, inflammation, blood sugars), diabetes, cancer and overweight (as reviewed by Kouris-Blazos and Belski (2016)). This may be due to the antioxidants (especially polyphenols), dietary fibre, essential fatty acids and isoflavones components of legumes, which have the potential of reducing the risk of cardiovascular disease by lowering blood cholesterol level (Moreno-Jiménez et al., 2015).
Legumes contains higher dietary fibre (6-40%) compared to cereals (2-7%) (Maphosa and Jideani, 2017). The relatively high soluble fibre contents in some legumes (Table 2) has the potential of lowering cholesterol by increasing the ratio of bile acid to salt excretion in faeces and by providing colonic bacteria with substrate, which is fermented anaerobically to Short-Chain Fatty Acid (SCFA) (acetate, propionate and butyrate) in the gut (Fabbri et al., 2016). Re-absorption of SCFA from the large intestine into the small intestine interferes with cholesterol production and thus, protects the cardiovascular system. Propionate interferes with endogenous cholesterol production, thereby, lowering serum cholesterol (Padhi and Ramdath, 2017). In vitro and in vivo studies have shown that butyrate can inhibit histone deacetylase and consequently, modulate the expression of genes involved in cancer cell proliferation, differentiation and apoptosis (Wang et al., 2013). In addition, saponins and phytosterols in legumes can help in decreasing the absorption of cholesterol from human gut (Rochfort and Panozzo, 2007).
Legumes micronutrients such as folate, selenium and bioactive phytochemicals (saponins, phytic acid, lectins and polyphenols) are promising agents against cancer. Legume consumption has been reported to provide protection against bowel, breast, prostate and colorectal cancers (Wang et al., 2013; Caan et al., 2011; Conroy et al., 2013). Evidence from observational and clinical studies have shown that polyphenolic phytochemicals such as phenolic acid and isoflavones from legumes especially soybeans can reduce the risk of breast cancer and its recurrence (Braakhuis et al., 2016; Nechuta et al., 2012).
Legumes are rich in isoflavones, which has protective effects against bone loss in postmenopausal women (Atteritano et al., 2009). Isoflavones improves endothelial function and reduce the progression of subclinical atherosclerosis. This indicates that legumes rich in isoflavone genistein such as soybeans can alleviate menopausal hot flashes, provide protective effects and improve the general wellbeing of postmenopausal women (Atteritano et al., 2009; Messina, 2014).
Epidemiological studies have proven that diet containing antioxidants such as legume can reduce the occurrence of degenerative diseases (Bouchenak and Lamri-Senhadji, 2013). Observational studies have also shown that regular consumption (>4 serving/week) of legumes can reduce cardiovascular disease by 11% and coronary heart disease by 22% (Padhi and Ramdath, 2017). This is probably due to high content of isoflavones in legumes. Numerous mechanistic studies reported that the capacity of isoflavones in preventing cardiovascular disease may be due to its ability to act similar to estrogens (Bouchenak and Lamri-Senhadji, 2013).
Legumes have the potential to reduce the risk of non-insulin dependent diabetes due to its low energy and glycaemic index (Dornbos and Mullen, 1992). Legumes contain high level of soluble fibre in addition to slow release carbohydrate which aid in glycemic control. In congruent with these properties of legumes, studies conducted with Chinese women that consume high amount of legume regularly showed that high intake of legumes can reduce the risk of Type 2 diabetes mellitus by 38-47% in women (Bouchenak and Lamri-Senhadji, 2013).
Legume plays important role in weight control and in preventing obesity. The benefit of legumes in weight management is associated with increased satiety and high nutrient, which decreases food intake and promote weight reduction by reducing accumulation of excessive adipose tissue (Padhi and Ramdath, 2017; Clark and Duncan, 2017). This is because legumes are rich in soluble fibre and resistance starch. When legumes are consumed, their carbohydrate forms viscous gel in the small intestine which delays gastric emptying and consequently delays digestion and thus causes delay in hunger (Howarth et al., 2001; McCrory et al., 2010). High fibre and protein and low glycaemic index of legumes also contribute to reduced hunger after a legume based diet (Gupta, 1987). This is in consistent with observational study that showed that beans consumers have lower body weight and waist circumference compared with non-consumers (Padhi and Ramdath, 2017).
In developing countries, legumes (mostly in the form of flour) are used to supplement cereal, root or tuber diets, however, the percentage of legumes used in supplementation is generally low (Bolarinwa et al., 2017; Eneche, 1999). Thus, this makes the amount of nutrients available for people’s consumption from the supplemented food low. In addition, legume flour has low protein digestibility compared to cooked legumes (Tuan and Phillips, 1991). On the other hand, if whole legumes are consumed, available nutrients will be much higher. However, since most whole legume-based diets required prolong cooking time until the legume becomes sufficiently soft and high cost of energy is required for this process, low income earners in developing countries cannot afford regular consumption of whole cooked legume seeds. To overcome this problem, the effect of different pre-treatment methods on legume cooking time has been investigated and reported. For instance, the use of different treatments such as soaking (in water, bicarbonate or salt mixture solutions), blanching (with chelating agents or enzymes) and decortication prior to cooking have been reported to reduce the cooking time of legumes by 25% to 80%, depending on the type of legume and the pre-treatment method employed (El‐Tabey Shehata, 1992). In addition, there is need for plant breeders to produce legume varieties (especially beans, cowpeas, soybeans, chickpeas) with softer seed coat and thus, short cooking times. This will further increase industrial utilization and food usage of legumes in developing countries.
Conclusion
Legumes are sources of protein, fat, carbohydrates and dietary fibre, with significant amounts of B-vitamins, minerals, antioxidants and high energetic value. This high nutritional profile of legume makes it an excellent crop for reducing protein-energy malnutrition (when paired with grains) and micronutrient deficiency, with a potential of improving the livelihoods of small holder farmers and increasing the life span of the low income earners in developing countries. Its drought resistance, soil enriching ability and ability to be processed into different forms and used for nutrient supplementation in cereal and root or tuber-based foods, all together placed it as a food and nutrition security crop in less developed countries. Future research should tend towards application of modified legume protein and starch as functional food ingredients, isolation of legume proteins and determination of their functional properties for possible domestic or industrial value, and development of new cheap technology to overcome the hard-to-cook phenomenon of legumes. There is also the need to explore more on the nutritional profile and anti-nutritional factors of less commonly consumed or underutilized legumes and their potential in food usage and industrial utilization for either food formulation, pharmaceutical ingredient or as biofuel.
Acknowledgement
Author I.F. Bolarinwa is grateful to Universiti Putra Malaysia (UPM) and the World Academy of Science (TWAS) for postdoctoral fellowship award, and to Ladoke Akintola University of Technology, Nigeria, for releasing her for the programme.
Author Details
1Faculty of Food Science and Technology, Universiti Putra Malaysia, 43400 UPM, Serdang, Selangor, Malaysia
2Department of Food Science and Engineering, Ladoke Akintola University of Technology, PMB 4000, Ogbomoso, Nigeria
References
Rights and permissions
Open Access: This article is licensed under a Creative Commons Attribution 4.0 International License, which permits use, sharing, adaptation, distribution and reproduction in any medium or format, as long as you give appropriate credit to the original author(s) and the source, provide a link to the Creative Commons license, and indicate if changes were made. The images or other third-party material in this article are included in the article’s Creative Commons license, unless indicated otherwise in a credit line to the material. If material is not included in the article’s Creative Commons license and your intended use is not permitted by statutory regulation or exceeds the permitted use, you will need to obtain permission directly from the copyright holder. To view a copy of this license, visit http://creativecommons.org/licenses/by/4.0/
Cite this Article
DOI: http://doi.org/10.19026/ajfst.17.6032
Sections